Abstract
An understanding of the response of multiaxially loaded concrete to the expansive reactions, such as alkali-silica reaction (ASR), remains a prerequisite to predict the long-term behavior of ASR-affected concrete structures, such as dams and containment structures. However, no suitable techniques exist to apply biaxial and triaxial stresses in concrete specimens, and to sustain the stresses for monitoring the long-term behavior of concrete. Consequently, state-of-the-art numerical modeling of concrete structures undergoing ASR relies on the experimental findings obtained from specimens with insufficient restraints. This paper presents the development of a new method to apply multiaxial (uniaxial, biaxial and triaxial) stresses. The stresses were applied to multiple concrete cube specimens, and were sustained for 1 year as ASR evolved in the specimens subjected to an accelerated condition of 50 °C temperature and close to 100 % relative humidity. The specimens were measured for longitudinal expansion in the three directions. The merit of the proposed method in the study of ASR is illustrated by presenting the results on the triaxial expansions of several unloaded and multiaxially loaded cube specimens until the exhaustion of the reaction. The results demonstrate a strong influence of multiaxial stress states on the ASR expansion of concrete.










Similar content being viewed by others
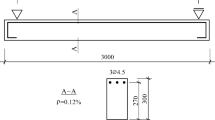
References
Popovic A, Panesar DK, Elgohary M (2002) Repair of the Gentilly-1 concrete containment structure. OECD-NEA workshop on the evaluation. Defects, repair criteria methods repair concrete structures nuclear power plants. OECD-NEA, Berlin, p 11
Saouma VE, Hariri-Ardebili MA (2014) A proposed aging management program for alkali silica reactions in a nuclear power plant. Nucl Eng Des 277:248–264
Leger P, Cote P, Tinawi R (1996) Finite element analysis of concrete swelling due to alkali-aggregate reactions in dams. Comput Struct 60:601–611
Multon S, Toutlemonde F (2006) Effect of applied stresses on alkali–silica reaction-induced expansions. Cem Concr Res 36:912–920
Huang M, Pietruszczak S (1999) Modeling of thermomechanical effects of alkali-silica reaction. J Eng Mech 125:476–485
Ulm F-J, Coussy O, Kefei L, Larive C (2000) Thermo-chemo-mechanics of ASR expansion in concrete structures. J Eng Mech 126:233–242
Grimal E, Sellier A, Le Pape Y, Bourdarot E (2008) Creep, shrinkage, and anisotropic damage in alkali-aggregate reaction swelling mechanism—Part I: a constitutive model. ACI Mater J 105:227–235
Grimal E, Sellier A, Le Pape Y, Bourdarot E (2008) Creep, shrinkage, and anisotropic damage in alkali-aggregate reaction swelling mechanism—Part II: identification of model parameters and application. ACI Mater J 105:236–242
Saouma V, Perotti L (2006) Constitutive model for alkali-aggregate reactions. ACI Mater J 103:194–202
Ferraris CF, Garboczi EJ, Davis FL, Clifton JR (1997) The effect of stress relaxation, self-desiccation, and water absorption on the alkali-silica reaction in low water/cement ratio mortars. Cem Concr Res 25:1553–1560
Kawamura M, Iwahori K (2004) ASR gel composition and expansive pressure in mortars under restraint. Cem Concr Compos 26:47–56
Berra M, Faggiani G, Mangialardi T, Paolini AE (2010) Influence of stress restraint on the expansive behaviour of concrete affected by ASR. Cem Concr Res 40:1403–1409
Dunant CF, Scrivener KL (2012) Effects of uniaxial stress on alkali–silica reaction induced expansion of concrete. Cem Concr Res 42:567–576
Larive C (1998) Combined contribution of experiments and modelling to the understanding of alkali-aggregate reaction and its mechanical consequences (PhD thesis; in: French). Ecole Nationale des Ponts et Chausses
Yurtdas I, Chen D, Hu DW, Shao JF (2013) Influence of alkali silica reaction (ASR) on mechanical properties of mortar. Constr Build Mater 47:165–174
Furr HL (1967) Creep tests of two-way prestressed concrete. ACI J 64:288–294
ASTM C1293 (2008) Standard test method for determination of length change of concrete due to alkali-silica reaction. ASTM International, West Conshohocken, PA
Fournier B, Rogers CA, Macdonald C (2012) Multilaboratory study of the concrete prism and accelerated mortar bar expansion tests with Spratt aggregate. 14th International Conference Alkali Aggregate Reaction, Texas, p 10
PCC Energy Group Collapsing pressure for tubing subject to external pressures. http://www.pccenergy.com/technical-information. Accessed 14 Jun 2015
Roscoe Moss Company Collapse strength of steel casing. http://roscoemoss.com/technical-resources/calculators/. Accessed 14 Jun 2015
Wang J (2009) Buckling behavior and design of steel liner encased in deep water tunnel under external hydrostatic pressure (PhD thesis). Waseda University
Petrowiki Strength of casing and tubing: elastic collapse. http://petrowiki.org/Strength_of_casing_and_tubing. Accessed 14 Jun 2015
ASTM A193 (2012) Standard specification for alloy-steel and stainless steel bolting for high temperature or high pressure service and other special purpose applications. ASTM International, West Conshohocken, PA
ASTM A194 (2012) Standard specification for carbon and alloy steel nuts for bolts for high pressure or high temperature service, or both. ASTM International, West Conshohocken, PA
CSA A23.1-09/A23.2-09 (2009) Concrete materials and methods of concrete construction/test methods and standard practices for concrete. Canadian Standards Association, Mississauga
CSA A23.3-04 (2004) Design of concrete structures. Canadian Standards Association, Mississauga
General Atomics (1988) Metals design handbook. San Diego, CA
Hall K, Tayabji S (2011) Coefficient of thermal expansion in concrete pavement design. ACPT TechBrief 1–6
Acknowledgements
The authors acknowledge the funding provided through a contract by Canadian Nuclear Safety Commission. The authors are grateful to the support provided by the technical staff of the Department of Civil Engineering at the University of Toronto. Also acknowledged is the help of summer students during preparatory work, casting and loading of cube specimens.
Author information
Authors and Affiliations
Corresponding author
Rights and permissions
About this article
Cite this article
Gautam, B.P., Panesar, D.K. A new method of applying long-term multiaxial stresses in concrete specimens undergoing ASR, and their triaxial expansions. Mater Struct 49, 3495–3508 (2016). https://doi.org/10.1617/s11527-015-0734-z
Received:
Accepted:
Published:
Issue Date:
DOI: https://doi.org/10.1617/s11527-015-0734-z