Abstract
The fatigue crack growth (FCG) tests were performed on a Ni–17Mo–7Cr base superalloy before and after thermal exposure to investigate the influence of microstructural variation on the alloy’s FCG behavior. It was observed that the original alloy consists of Ni matrix and MoC particles. When the alloy was aged at 1350 °C for 0.5 h, the MoC melts together with circumambient Ni matrix, forming a eutectic–like structure. The microstructural analysis shows that, for original alloy, the crack basically propagates in the matrix. When it encounters the particles, the crack meanders to avoid them, creating a linear d a /d N–Δ K curve. As the crack approaches the eutectic-like structures within the aged alloy, however, it cuts through them but circumvents tiny particles within the structures, producing torturous crack paths and waved d a /d N–Δ K curve. The aged alloy has a higher FCG resistance in contrast to original alloy.















Similar content being viewed by others
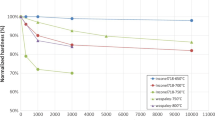
References
Y. Mutoh, A.A. Korda, Y. Miyashita, and T. Sadasue: Stress shielding and fatigue crack growth resistance in ferritic–pearlitic steel. Mater. Sci. Eng., A 468–470, 114 (2007).
M. Hashim, K.E.S.R. Babu, M. Duraiselvam, and H. Natu: Improvement of wear resistance of Hastelloy C–276 through laser surface melting. Mater. Des. 46, 546 (2013).
Z.F. Xu, L. Jiang, J.S. Dong, Z.J. Li, and X.T. Zhou: The effect of silicon on precipitation and decomposition behaviors of M6C carbide in a Ni–Mo–Cr superalloy. J. Alloys. Compd. 620, 197 (2015).
W. Abuzaid, H. Sehitoglu, and J. Lambros: Plastic strain localization and fatigue micro–crack formation in Hastelloy X. Mater. Sci. Eng., A 561, 507 (2013).
H.E. McCoy: Influence of Several Metallurgical Variables on the Tensile Properties of Hastelloy N, ORNL–3661 (Oak Ridge National Laboratory, Oak Ridge, 1964); pp. 12, 54.
R.B. Briggs: Molten–salt Reaction Program Semiannual Progress Report, ORNL–3872 (Oak Ridge National Laboratory, Oak Ridge, 1965); p. 95.
Y.M. He, J.G. Yang, S.J. Chen, and Z.L. Gao: Influence of simulated heat–affected zone thermal cycle treatment on mechanical performances and microstructural stability of Ni–17Mo–7Cr based superalloy. Vacuum 26, 125 (2016).
M.A. Sutton, B.C. Yang, A.P. Reynolds, and J.H. Yan: Banded microstructure in 2024–T351 and 2524–T351 aluminum friction stir welds: Part II. Mechanical characterization. Mater. Sci. Eng., A 364, 66 (2004).
H.L. Ewalds and R.J.H. Wanhill: Fracture Mechanics (Edward Arnold Publication, London, 1986); pp. 172, 276.
L. Wang, S. Wang, X. Song, Y. Liu, and G.H. Xu: Effects of precipitated phases on the crack propagation behavior of a Ni–based superalloy. Int. J. Fatigue 62, 210 (2014).
A.A. Korda, Y. Miyashita, Y. Mutoh, and T. Sadasue: Fatigue crack growth behavior in ferritic–pearlitic steels with networked and distributed pearlite structures. Int. J. Fatigue 29, 1140 (2007).
S.L. Zheng, Q. Yu, and Y.Y. Jiang: An experimental study of fatigue crack propagation in extruded AZ31B magnesium alloy. Int. J. Fatigue 47, 174 (2013).
X.D. Lu and H.M. Wang: High–temperature phase stability and tribological properties of laser clad Mo2Ni3Si/NiSi metal silicide coatings. Acta Mater. 52, 5147 (2004).
S. Floreen, G.E. Fuchs, and W.J. Yang: The metallurgy of alloy 625. In Superalloys 718, 625, 706 and Various Derivatives, E.A. Loria, ed. (The Minerals, Metals & Materials Society, Pittsburgh, 1994); pp. 13–37.
Z.Q. Song, Q. He, E. Ma, and J. Xu: Fatigue endurance limit and crack growth behavior of a high–toughness Zr61Ti2Cu25Al12 bulk metallic glass. Acta Mater. 99, 165 (2015).
M.F. Guan and H. Yu: Fatigue crack growth behaviors in hot–rolled low carbon steels: A comparison between ferrite–pearlite and ferrite–bainite microstructures. Mater. Sci. Eng., A 559, 875 (2013).
M.J. Li, Q.L. Pan, Y. Wang, and Y.J. Shi: Fatigue crack growth behavior of Al–Mg–Sc alloy. Mater. Sci. Eng., A 598, 350 (2014).
P.H. Ma, L.H. Qian, J.Y. Meng, S. Liu, and F.C. Zhang: Influence of Al on the fatigue crack growth behavior of Fe–22Mn–(3Al)–0.6C TWIP steels. Mater. Sci. Eng., A 645, 136 (2015).
D.L. Chen, M.C. Chaturvedi, N. Goel, and N.L. Richards: Fatigue crack growth behavior of X2095 Al–Li alloy. Int. J. Fatigue 21, 1079 (1999).
A.A. Korda, Y. Mutoh, Y. Miyashita, T. Sadasue, and S.L. Mannan: In situ observation of fatigue crack retardation in banded ferrite–pearlite microstructure due to crack branching. Scr. Mater. 54, 1835 (2006).
S. Suresh: Micro–mechanisms of fatigue crack growth retardation following overloads. Eng. Fract. Mech. 18 (3), 577 (1983).
Y.M. He, J.G. Yang, W. Fu, L.M. Wang, and Z.L. Gao: An analysis of formation mechanism and nano–scale hardness of the laser–induced coating on Ni17Mo7Cr based superalloy. J. Alloys Compd. 673, 8 (2016).
D.H. Jeong, M.J. Choi, M. Goto, H.C. Lee, and S. Kim: Effect of service exposure on fatigue crack propagation of Inconel 718 turbine disc material at elevated temperatures. Mater. Charact. 95, 232 (2014).
R. Jiang, N. Karpasitis, N. Gao, and P.A.S. Reed: Effects of microstructures on fatigue crack initiation and short crack propagation at room temperature in an advanced disc superalloy. Mater. Sci. Eng., A 641, 148 (2015).
ACKNOWLEDGMENTS
The financial support of National Natural Science Foundation of China under Grant Nos. 51405439 and 51475426 is highly acknowledged.
Author information
Authors and Affiliations
Corresponding authors
Rights and permissions
About this article
Cite this article
He, Y., Yang, J. & Zheng, S. Microstructure dependent fatigue crack growth characteristics in the Ni–17Mo–7Cr base superalloy before and after thermal exposure. Journal of Materials Research 31, 3880–3890 (2016). https://doi.org/10.1557/jmr.2016.424
Received:
Accepted:
Published:
Issue Date:
DOI: https://doi.org/10.1557/jmr.2016.424