Abstract
Because of the depletion of fossil fuels and because of its increasing cost, waste has been used as alternative fuels in cement rotary kilns for several years. In order to fulfil the requirements of environmental protection and quality of the final product, it is necessary to understand and quantify the different processes occurring in the kiln. The aim of our work is to develop a mathematical model of the processes occurring in the kiln. This model will rely on the coupling between a CFD model and homemade software. More precisely, the CFD model, which will be fully three-dimensional will account for the homogeneous processes taking place in the freeboard of the bed of material being processed. This bed of material will be at the center of the second model which will represent it as a 1D plug flow reactor. In the present work, we focus on this 1D model. We first give insights on the main assumptions on which the model relies, and information on the reaction pathway leading to the production of cement. Indeed, it is considered that the bed is composed of a mixture of CaCO3, MgCO3, Al2O3, SiO2, Fe2O3, MgO, CaO, C2S, C3A, C4AF and C3S undergoing thermochemical transformation. The bed under consideration is also composed of biomass (agricultural residues). During its transformation (pyrolysis, combustion of volatiles, combustion of the pyrolysis residue), this material contributes to thermal equilibrium of the reactor, by carrying the energy associated to its complete combustion. In this paper, the different equations that translate into mathematical formalism the processes of transport of the bed as well as mass and energy balance are also presented. The results show that the cement obtained complies with the requirements of Portland cements (73.06% of Silicates and 18.76% of Aluminates), the conversion of the biomass is total (100%), and the specific energy consumption is almost in conformity with the values of literature.
Graphic Abstract














Similar content being viewed by others
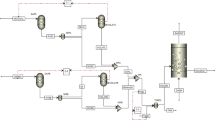
Abbreviations
- AE:
-
Algebraic equation
- CFD:
-
Computational fluid dynamics
- NCELL:
-
Number of cellules (numerical parameter)
- NESFASOL:
-
Number of species of solid meal (flour)
- NESDEC:
-
Number of species of waste (biomass)
- NESGAZ:
-
Number of gas species
- ODE:
-
Ordinary differential equation
- \(A_{lit}\) :
-
Transverse surface of the bed (m2)
- \(A\) :
-
Pre-exponential factor (s−1)
- \(c_{p}\) :
-
Specific heat (J kg−1 K−1)
- \(d_{four}\) :
-
Diameter of rotary kiln (m)
- \(d_{dec}\) :
-
Diameter of biomass particle (m)
- \(Ea\) :
-
Activation energy (J mol−1)
- \(e_{refrac}\) :
-
Refractory thickness (m)
- \(Fr\) :
-
Froude number
- \(g\) :
-
Gravity acceleration (m s−2)
- \(h_{f,i}^{0}\) :
-
Enthalpy of formation of the species I (J kg−1)
- \(h_{lit}\) :
-
Enthalpy of the bed (J kg−1)
- \(H_{lit}\) :
-
Bed height (m)
- \(h\) :
-
Heat exchange coefficient (W m−2 K−1)
- \(k\) :
-
Speed constant (s−1)
- \(l_{lit}\) :
-
Width of the bed (m)
- \(L_{four}\) :
-
Kiln length (m)
- \(M_{i}\) :
-
Molar mass of the species i (kg mol−1)
- \(\dot{m}\) :
-
Mass flow (kg s−1)
- \(N_{z}\) :
-
Number of waste particles (m−1)
- \(n_{four}\) :
-
Speed of rotation of the kiln (tr s−1)
- \(q_{v}\) :
-
Volume flow rate (m3 s−1)
- \(r\) :
-
Chemical reaction rate (kg m−2 s−1)
- \(R_{k}^{Fa,Fa}\) :
-
Specific net rate of production / disappearance of k species of flour (kg m−3 s−1)
- \(R_{q}^{dec,dec}\) :
-
Specific net rate of production/disappearance of species q of waste (kg m−3 s−1)
- \(R_{b}^{gaz,gaz}\) :
-
Specific net rate of production of species b associated with the homogeneous reactions in the gas phase (kg m−3 s−1)
- \(R_{b}^{fa,gaz}\) :
-
Specific net rate of production of species b by the decomposition reactions of flour (kg m−3 s−1)
- \(R_{b}^{dec,gaz}\) :
-
Specific net rate of production of species b gas by decomposition reactions of waste (kg m−3 s−1)
- \(S\) :
-
Surface (m2)
- \(S_{trans}\) :
-
Cross-sectional area of the kiln wall (m2)
- \(\left[ { i } \right]\) :
-
Molar/mass concentration
- \(T\) :
-
Temperature (K)
- \(u\) :
-
Speed (m s−1)
- \(y_{k}^{fa}\) :
-
Mass fraction of k species in meal (flour)
- \(y_{fa}^{lit}\) :
-
Mass fraction of the flour in the bed
- \(y_{q}^{dec}\) :
-
Mass fraction of species q in waste
- \(y_{dec}^{lit}\) :
-
Mass fraction of waste in the bed
- \(y_{b}^{gaz}\) :
-
Mass fraction of species b in gas
- \(y_{gaz}^{lit}\) :
-
Mass fraction of gas in the bed
- \(\delta_{four}\) :
-
Tilting of the kiln (rad)
- \(\delta_{lit}\) :
-
Angle of interception of the bed (rad)
- \(\varepsilon\) :
-
Emissivity (–)
- \(\lambda\) :
-
Thermal conductivity (W m−1 K−1)
- \(\rho\) :
-
Volume mass (kg m−3)
- \(\varphi_{dyn}\) :
-
Dynamic angle of repose of the bed (rad)
- \(\varphi\) :
-
Heat exchange flow (W)
- \(\omega\) :
-
Angular velocity (rad s−1)
- \(0\) :
-
Initial condition/kiln inlet
- \(Ashes\) :
-
Ashes
- \(Char,pyro\) :
-
Char pyrolysis
- \(dec\) :
-
Waste
- \(decouv\) :
-
Open wall
- \(ext\) :
-
Outside environment
- \(fa\) :
-
Meal
- \(four\) :
-
Kiln or wall of the kiln
- \(free\) :
-
Free
- \(free,lit\) :
-
Freeboard gas—bed of solids
- \(four,lit\) :
-
Covered wall of the kiln—bed of solids
- \(four,ext\) :
-
Outer wall of the kiln—outside environment
- \(gaz\) :
-
Gas
- \(gazFB\) :
-
Freeboard gas
- \(hum\) :
-
Humidity
- \(lit\) :
-
Bed of solids
- \(Org. Mat.\) :
-
Organic matter
- C:
-
CaO—calcium oxide or lime
- S:
-
SiO2—silicon dioxide or Silica
- A:
-
Al2O3—alumina
- F:
-
Fe2O3—iron oxide III
References
Martínez, J.D., Puy, N., Murillo, R., García, T., Navarro, M.V., Mastral, A.M.: Waste tyre pyrolysis: a review. Renew. Sustain. Energy Rev. 23, 179–213 (2013). https://doi.org/10.1016/j.rser.2013.02.038
Madlool, N.A., Saidur, R., Rahim, N.A., Islam, M.R., Hossian, M.S.: An exergy analysis for cement industries: an overview. Renew. Sustain. Energy Rev. 16, 921–932 (2012). https://doi.org/10.1016/j.rser.2011.09.013
Mungyeko Bisulandu, B.-J.R., Pongo Pongo, C.: Les énergies renouvelables face à l’épuisement des énergies fossiles: Utilisation et Valorisation des déchets dans les fours de cimenterie. In: 7éme Édition COFRET, Paris, France, pp. 905–918 (2014)
Kääntee, U., Zevenhoven, R., Backman, R., Hupa, M.: Cement manufacturing using alternative fuels and the advantages of process modelling. Fuel Process. Technol. 85, 293–301 (2004). https://doi.org/10.1016/S0378-3820(03)00203-0
Mokrzycki, E., Uliasz-Bocheńczyk, A., Sarna, M.: Use of alternative fuels in the Polish cement industry. Appl. Energy 74, 101–111 (2003). https://doi.org/10.1016/S0306-2619(02)00136-8
Kaddatz, K.T., Rasul, M.G., Rahman, A.: alternative fuels for use in cement kilns: process impact modelling. Procedia Eng. 56, 413–420 (2013). https://doi.org/10.1016/j.proeng.2013.03.141
Di Blasi, C.: Dynamic behaviour of stratified downdraft gasifiers. Chem. Eng. Sci. 55(2000), 2931–2944 (2000)
Aranda Usón, A., López-Sabirón, A.M., Ferreira, G., Llera Sastresa, E.: Uses of alternative fuels and raw materials in the cement industry as sustainable waste management options. Renew. Sustain. Energy Rev. 23, 242–260 (2013). https://doi.org/10.1016/j.rser.2013.02.024
(CINAT) Cimenterie Nationale, Données techniques (2010)
Çamdali, Ü., Eri̇şen, A., Çelen, F.: Energy and exergy analyses in a rotary burner with pre-calcinations in cement production. Energy Convers. Manag. 45, 3017–3031 (2004). https://doi.org/10.1016/j.enconman.2003.12.002
Chatterjee, A.K.: Chemistry and engineering of the clinkerization process — Incremental advances and lack of breakthroughs. Cem. Concr. Res. 41, 624–641 (2011). https://doi.org/10.1016/j.cemconres.2011.03.020
Ariyaratne, W.K.H., Malagalage, A., Melaaen, M.C., Tokheim, L.-A.: CFD modelling of meat and bone meal combustion in a cement rotary kiln: investigation of fuel particle size and fuel feeding position impacts. Chem. Eng. Sci. 123, 596–608 (2015). https://doi.org/10.1016/j.ces.2014.10.048
Babler, M.U., Phounglamcheik, A., Amovic, M., Ljunggren, R., Engvall, K.: Modeling and pilot plant runs of slow biomass pyrolysis in a rotary kiln. Appl. Energy. (2017). https://doi.org/10.1016/j.apenergy.2017.06.034
Nielsen, A.R.: Combustion of large solid fuels in cement rotary kilns: Ph.D. Thesis, Department of Chemistry, Technical University of Denmark, Kgs. Lyngby (2012). https://www.forskningsdatabasen.dk/en/catalog/2185763052
Boateng, A.A., Barr, P.V.: A thermal model for the rotary kiln including heat transfer within the bed. Int. J. Heat Mass Transf. 39, 2131–2147 (1996)
Marias, F.: A model of a rotary kiln incinerator including processes occurring within the solid and the gaseous phases. Comput. Chem. Eng. 27, 813–825 (2003). https://doi.org/10.1016/S0098-1354(02)00268-5
Mujumdar, K.S., Ranade, V.V.: Simulation of rotary cement kilns using a one-dimensional model. Chem. Eng. Res. Des. 84, 165–177 (2006). https://doi.org/10.1205/cherd.04193
Darabi, P.: A Mathematical Model for Cement Kilns. University of British Columbia, Vancouver (2007)
Spang, H.A.: A dynamic model of a cement kiln. Automatica 8, 309–323 (1972). https://doi.org/10.1016/0005-1098(72)90050-7
Chen, L., Dupont, C., Salvador, S., Grateau, M., Boissonnet, G., Schweich, D.: Experimental study on fast pyrolysis of free-falling millimetric biomass particles between 800°C and 1000°C. Fuel 106, 61–66 (2013). https://doi.org/10.1016/j.fuel.2012.11.058
Efika, E.C., Onwudili, J.A., Williams, P.T.: Products from the high temperature pyrolysis of RDF at slow and rapid heating rates. J. Anal. Appl. Pyrolysis. 112, 14–22 (2015). https://doi.org/10.1016/j.jaap.2015.01.004
Lopez, G., Alvarez, J., Amutio, M., Mkhize, N.M., Danon, B., van der Gryp, P., Görgens, J.F., Bilbao, J., Olazar, M.: Waste truck-tyre processing by flash pyrolysis in a conical spouted bed reactor. Energy Convers. Manag. 142, 523–532 (2017). https://doi.org/10.1016/j.enconman.2017.03.051
Arthur, J.R.: Reactions between carbon and oxygen. Trans. Faraday Soc. 47, 164 (1951). https://doi.org/10.1039/tf9514700164
Bernada, P., Marias, F., Deydier, A., Couture, F., Fourcault, A.: Modelling of a traveling bed WASTE gasifier. Waste Biomass Valoriz. 3, 333–353 (2012). https://doi.org/10.1007/s12649-012-9115-9
Marias, F., Benzaoui, A., Vaxelaire, J., Gelix, F., Nicol, F.: Fate of nitrogen during fluidized incineration of sewage sludge. Estimation of NO and N2O content in the exhaust gas. Energy Fuels 29, 4534–4548 (2015). https://doi.org/10.1021/acs.energyfuels.5b01109
Marias, F., Puiggali, J.-R.: Simulation numérique d’un brûleur industriel. Analyse qualitative des effets de swirl sur l’écoulement et sur la production de polluants. Int. J. Therm. Sci. 39, 249–264 (2000). https://doi.org/10.1016/S1290-0729(00)00243-X
Boateng, A.A., Barr, P.V.: Modelling of particle mixing and segregation in the transverse plane of a rotary kiln. Chem. Eng. Sci. 51, 4167–4181 (1996)
Werther, J., Saenger, M., Hartge, E.-U., Ogada, T., Siagi, Z.: Combustion of agricultural residues. Prog. Energy Combust. Sci. 26, 1–27 (2000). https://doi.org/10.1016/S0360-1285(99)00005-2
Menard, Y.: Modélisation de l’incinération sur grille d’ordures ménagères et approche thermodynamique du comportement des métaux lourds. Institut National Polytechnique de Lorraine (2003)
Teislev, B.: Simulation of the biomass updraft gasifier (2006)
Vàhl, L., Kingma, W.G.: Transport of solids through horizontal rotary cylinders. Chem. Eng. Sci. 1, 253–258 (1952). https://doi.org/10.1016/0009-2509(52)87018-6
Kramers, H., Croockewit, P.: The passage of granular solids through inclined rotary kilns. Chem. Eng. Sci. 1, 259–265 (1952). https://doi.org/10.1016/0009-2509(52)87019-8
Marias, F., Roustan, H., Pichat, A.: Modelling of a rotary kiln for the pyrolysis of aluminium waste. Chem. Eng. Sci. 60, 4609–4622 (2005). https://doi.org/10.1016/j.ces.2005.03.025
Patisson, F., Lebas, E., Hanrot, F., Ablitzer, D., Houzelot, J.-L.: Coal pyrolysis in a rotary kiln: Part I. Model of the pyrolysis of a single grain. Metall. Mater. Trans. B. 31, 381–390 (2000). https://doi.org/10.1007/s11663-000-0056-5
Patisson, F., Lebas, E., Hanrot, F., Ablitzer, D., Houzelot, J.-L.: Coal pyrolysis in a rotary kiln: Part II. Overall model of the furnace. Metall. Mater. Trans. B. 31, 391–402 (2000)
Ginsberg, T., Modigell, M.: Dynamic modelling of a rotary kiln for calcination of titanium dioxide white pigment. Comput. Chem. Eng. 35, 2437–2446 (2011). https://doi.org/10.1016/j.compchemeng.2011.03.029
Lybaert, P.: Contribution à l’étude du transfert de chaleur entre un matériau particulaire et la paroi dans les échangeurs rotatifs indirects, Faculté Polytechnique de Mons (1985)
Kohlhaas, B.: Otto Labahn, Cement Engineers Handbook, 6th edn. Bauverlag GMBH, Berlin (1983)
Csernyei, C., Straatman, A.G.: Numerical modeling of a rotary cement kiln with improvements to shell cooling. Int. J. Heat Mass Transf. 102, 610–621 (2016). https://doi.org/10.1016/j.ijheatmasstransfer.2016.06.058
Mastorakos, E., Massias, A., Tsakiroglou, C.D., Goussis, D.A., Burganos, V.N., Payatakes, A.C.: CFD predictions for cement kilns including Flame modelling, heat transfer and clinker chemistry. Appl. Math. Model. 23, 55–76 (1999)
Pongo Pongo, C.: Caractéristiques des produits Cinat (2012)
Lixhe CBR: Fiche technique-Usine de Lixhe (2012)
Acknowledgements
We wish to acknowledge Kongo University in the Democratic Republic of Congo for funding our research work. We also thank the managers of congolese cement plants, CINAT and CILU, for the technical data they provided us.
Author information
Authors and Affiliations
Corresponding author
Additional information
Publisher's Note
Springer Nature remains neutral with regard to jurisdictional claims in published maps and institutional affiliations.
Rights and permissions
About this article
Cite this article
Mungyeko Bisulandu, BJ.R., Marias, F. Modeling of the Thermochemical Conversion of Biomass in Cement Rotary Kiln. Waste Biomass Valor 12, 1005–1024 (2021). https://doi.org/10.1007/s12649-020-01001-9
Received:
Accepted:
Published:
Issue Date:
DOI: https://doi.org/10.1007/s12649-020-01001-9