Abstract
Numerical experiments to understand the resonant acoustic response of a subsonic jet impinging on the mouth of a tube, known as the Hartmann whistle configuration, were performed as large-eddy simulations. The tube length was chosen so that its fundamental duct mode, for one end closed and one end open, would match the dominant mode in the exciting jet. When the tube mouth was placed in the path of a regular stream of vortex rings, formed by the instability of the jet’s bounding shear layer, a strong resonant, tonal response (whistling) was obtained. At three diameters from the jet, OASPL was 150–160 dB. A tube with a thicker lip generated a louder response. When the tube was held closer to the nozzle exit, the impinging unsteady shear layer could not provoke any significant resonance. The simulations reveal that the tonal response of a Hartmann whistle operating in subsonic mode is significant.



Similar content being viewed by others
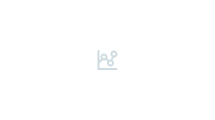
Abbreviations
- A :
-
area of the tube inlet (\(\hbox {m}^2\))
- a :
-
speed of sound (\(\hbox {ms}^{-1}\))
- f :
-
frequency (Hz)
- L :
-
length of the tube (m)
- M :
-
Mach number
- p :
-
static pressure (\(\hbox {N}/\hbox {m}^2\))
- \(p_0\) :
-
total pressure (\(\hbox {N}/\hbox {m}^2\))
- R :
-
nozzle pressure ratio (\(p_0/p_{\infty }\))
- Re:
-
Reynolds number
- \(r_j\) :
-
jet radius (m)
- S :
-
stand-off distance (m)
- St:
-
Strouhal number (\(\textit{fU}_j/2r_0\))
- \(U_j\) :
-
jet velocity at nozzle exit (\(\hbox {ms}^{-1}\))
- x :
-
reduced length (no unit)
- \(x_{in}\) :
-
value of variable x at nozzle exit
- \(x_\infty \) :
-
value of variable x at ambient conditions
- \(\rho \) :
-
density (\(\hbox {kg}/\hbox {m}^3\))
- \(\theta \) :
-
polar angle (degrees)
- \(\phi _{ij}(f)\) :
-
cross-power spectral density (\(\hbox {Pa}^2/\hbox {Hz}\))
- \(\phi _{ii}(f)\) :
-
auto-power spectral density (\(\hbox {Pa}^2/\hbox {Hz}\))
- \(\tau _{ij}\) :
-
shear stress tensor
- \(\mu \) :
-
dynamic viscosity (\(\hbox {Nsm}^{-2}\))
- PSD:
-
power spectral density (\(\hbox {Pa}^2/\hbox {Hz}\))
- SPL:
-
sound pressure level (dB), \(\hbox {p}_{ref} = 20 \times 10^{-6}\ \hbox {Pa}\)
- OASPL:
-
overall sound pressure level (dB)
References
Hartmann J 1922 On a new method for the generation of sound-waves. Phys. Rev. 20(6): 719
Raman G and Srinivasan K 2009 The powered resonance tube: from Hartmann’s discovery to current active flow control applications. Prog. Aerosp. Sci. 45(4): 97–123
Chang K S, Kim K H and Iwamoto J 1996 A study on the Hartmann–Sprenger tube flow driven by a sonic jet. A study on the Hartmann–Sprenger tube flow driven by a sonic jet 13(3): 173–182
Hamed A, Das K and Basu D 2002 Numerical simulation of unsteady flow in resonance tube. AIAA Paper 1118:2002
Cain A B, Kerschen E J and Raman G 2002 Simulation of acoustic characteristics and mechanisms of powered resonance tubes. In: Proceedings of the 8th AIAA/CEAS Aeroacoustics Conference and Exhibit, Breckenridge, CO
Hamed A, Das K and Basu D 2003 Numerical simulation and parametric study of Hartmann–Sprenger tube based powered device. In: Proceedings of the 41st Aerospace Sciences Meeting and Exhibit, Reno, NV, USA, pp. 6–9
Hamed A, Das K, and Basu D 2003 Characterization of powered resonance tube for high frequency actuaton. FEDSM2003-45472
Braud C and Dyment A 2012 Model of an impulsive subsonic jet actuator for flow control applications, Phys. Fluids 24(4): 047102
Sprenger H 1954 On thermal effects in resonance tubes. Mitt. Inat. Aerodyn. ETH 21: 18–24
Brocher E, Maresca C and Bournay M H 1970 Fluid dynamics of the resonance tube. J. Fluid Mech. 43:369–384
Sarohia V and Back L H 1979 Experimental investigation of flow and heating in a resonance tube. J. Fluid Mech. 94: 649–672
Brocher E and Duport E 1988 Resonance tubes in a subsonic flowfield. AIAA J. 26(5): 548–552
Mathew J, Lechner R, Foysi H, Sesterhenn J and Friedrich R 2003 An explicit filtering method for large eddy simulation of compressible flows, Phys. Fluids 15(8):2279–2289
Visbal M R and Rizzetta D P 2002 Large-eddy simulation on curvilinear grids using compact differencing and filtering Schemes, ASME J. Fluids Eng. 124(December): 836
Bogey C and Bailly C 2006 Computation of a high Reynolds number jet and its radiated noise using large eddy simulation based on explicit filtering. Comput. Fluids 35(10): 1344–1358
Hixon R and Turkel E 2000 Compact implicit MacCormack-type schemes with high accuracy. J. Comput. Phys. 158(1): 51–70
Lele S K 1992 Compact finite difference schemes with spectral-like resolution. J. Comput. Phys. 103(1):16–42
Poinsot T J and Lele S K 1992 Boundary conditions for direct simulations of compressible viscous flows. J. Comput. Phys., 101(1): 104–129
Ganesh S 2016 Large eddy simulation of free and impinging subsonic jets and their sound fields. PhD Thesis, Department of Aerospace Engineering, Indian Institute of Science
Bodony D J and Lele S K 2004 Jet noise prediction of cold and hot subsonic jets using large-eddy simulation. In: Proceedings of the 10th AIAA/CEAS Aeroacoustics Conference. Paper AIAA-2004-3022
Bogey C and Bailly C 2006 Large eddy simulations of transitional round jets: influence of the Reynolds number on flow development and energy dissipation. Phys. Fluids 18(6): 065101
Panchapakesan N R and Lumley J L 1993 Turbulence measurements in axisymmetric jets of air and helium. Part 1. Air jet. J. Fluid Mech. 246:197–223
Zaman K B M Q 1998 Asymptotic spreading rate of initially compressible jets—experiment and analysis. Phys. Fluids 10(10): 2652
Varadharajan R 2016 LES study of free jets and jets impinging on cuboidal cavity. Master Thesis, Department of Aerospace Engineering, Indian Institute of Science
Varadharajan R 2017 Reynolds number effects on transition, turbulence intensity and axial-velocity decay rate of turbulent round jets. arXiv preprint arXiv:1708.03140
Freund J B 2001 Noise sources in a low-Reynolds-number turbulent jet at Mach 0.9. J. Fluid Mech. 438: 277–305
Narayanan S, Bhave P, Srinivasan K, Ramamurthi K and Sundararajan T 2009 Spectra and directivity of a Hartmann whistle, J. Sound Vib. 321(3): 875–892
Acknowledgements
We thank Dr Santosh Hemachandra, Aerospace Engineering, IISc, for helpful discussions of this work.
Author information
Authors and Affiliations
Corresponding author
Rights and permissions
About this article
Cite this article
Varadharajan, R., Kamin, M., Ganesh, S. et al. On jet instability modes of a subsonic Hartmann whistle. Sādhanā 43, 140 (2018). https://doi.org/10.1007/s12046-018-0921-z
Received:
Revised:
Accepted:
Published:
DOI: https://doi.org/10.1007/s12046-018-0921-z