Abstract
Purpose
Ferrous iron (Fe(II)) oxidation and nitrate (NO3 −) reduction are commonly observed in environments with denitrifying bacteria. The intermediate nitrite (NO2 −) from denitrification can chemically oxidize Fe(II). However, it is difficult to distinguish how chemical and biological reactions are involved. Pseudomonas stutzeri LS-2, a denitrifying bacterium isolated from paddy soil in southern China, was used in this study to investigate the chemical and biological reactions contributing to Fe(II) oxidation and NO3 − reduction under denitrifying conditions.
Materials and methods
Concentrations of dissolved Fe(II), NO3 −, NO2 −, and nitrous oxide (N2O) over time were quantified to investigate the kinetics of Fe(II) oxidation and NO3 −/NO2 − reduction in different treatments (i.e., microbial treatments: Cell + NO3 − and Cell + NO2 −, chemical treatment: Fe(II) + NO2 −, and combined treatments: LS-2 + Fe(II) + NO3 − and LS-2 + Fe(II) + NO2 −). Stable isotope fractionations of δ15N-N2O in different treatments were also determined over time. Fe(III) minerals and cell-mineral precipitates formed due to Fe(II) oxidation after 6 days of incubation were characterized using X-ray diffraction (XRD), scanning electron microscopy (SEM), and transmission electron microscopy (TEM).
Results and discussion
P. stutzeri LS-2 could completely reduce NO3 − or NO2 − within 2 days in the microbial treatment of Cell + NO3 − or Cell + NO2 −. The presence of Fe(II) resulted in a decrease of NO3 − or NO2 − reduction rates and an increase in the amount of nitrous oxide (N2O) production in the combined treatments of Cell + Fe(II) + NO3 − and Cell + Fe(II) + NO2 −. Fe(II) oxidation was only observed in the two combined treatments and the chemical treatment of Fe(II) + NO2 −. Lepidocrocite was formed due to Fe(II) oxidation after 6 days of incubation, which fully covered the bacterial cell surfaces in both combined treatments. Encrustation occurred in the periplasm and on the cell surface. The δ15N-N2O were 7.8 to − 10‰ in both microbial treatments during incubation, while those were − 23 to − 15‰ in the Fe(II) + NO2 − and Cell + Fe(II) + NO2 − treatments. In the Cell + Fe(II) + NO3 − treatment, however, the δ15N in N2O were − 37 to − 25‰, which were different from the microbial and chemical treatments. This difference is probably due to the accelerated reaction between Fe(II) and NO3 −/NO2 − by lepidocrocite.
Conclusions
Our results indicate that once NO3 − was reduced to NO2 − by the denitrifying bacterium P. stutzeri LS-2, the NO2 − chemically reacted with Fe(II), and the concomitant Fe(III) oxide formation and cell encrustation led to an inhibition to denitrification. The stable isotope fractionation technique in combination with the transformation kinetics analyses is useful to distinguish the chemical and biological reactions involved in Fe(II) oxidation and nitrate reduction by denitrifying bacteria.







Similar content being viewed by others
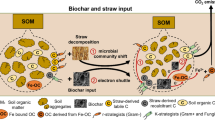
References
Barford CC, Montoya JP, Altabet MA, Mitchell R (1999) Steady-state nitrogen isotope effects of N2 and N2O production in Paracoccus denitrificans. Appl Environ Microbiol 65(3):989–994
Bruce RA, Achenbach LA, Coates JD (1999) Reduction of (per)chlorate by a novel organism isolated from paper mill waste. Environ Microbiol 1(4):319–329. https://doi.org/10.1046/j.1462-2920.1999.00042.x
Byrne-bailey KG, Weber KA, Chair AH, Bose S, Knox T, Spanbauer TL, Chertkov O, Coates JD (2010) Completed genome sequence of the anaerobic iron-oxidizing bacterium Acidovorax ebreus strain TPSY. J Bacteriol 192(5):1475–1476. https://doi.org/10.1128/JB.01449-09
Carlson HK, Clark IC, Blazewicz SJ, Iavarone AT, Coates JD (2013) Fe(II) oxidation is an innate capability of nitrate-reducing bacteria that involves abiotic and biotic reactions. J Bacteriol 195(14):3260–3268. https://doi.org/10.1128/JB.00058-13
Chakraborty A, Roden EE, Schieber J, Picardal F (2011) Enhanced growth of Acidovorax sp. strain 2AN during nitrate-dependent Fe(II) oxidation in batch and continuous-flow systems. Appl Environ Microbiol 77(24):8548–8556. https://doi.org/10.1128/AEM.06214-11
Chaudhuri SK, Lack JG, Coates JD (2001) Biogenic magnetite formation through anaerobic biooxidation of Fe(II). Appl Environ Microbiol 67(6):2844–2848. https://doi.org/10.1128/AEM.67.6.2844-2848.2001
Cooper DC, Picardal FW, Schimmelmann A, Coby AJ (2003) Chemical and biological interactions during nitrate and goethite reduction by Shewanella putrefaciens 200. Appl Environ Microbiol 69(6):3517–3525. https://doi.org/10.1128/AEM.69.6.3517-3525.2003
Delong EF (1992) Archaea in coastal marine environments. Proc Nat Acad Sci US 89(12):5685–5689. https://doi.org/10.1073/pnas.89.12.5685
Heil J, Wolf B, Brüggemann N, Emmenegger L, Tuzson B, Vereecken H, Mohn J (2014) Site-specific 15N isotopic signatures of abiotically produced N2O. Geochim Cosmochim Acta 139:72–82. https://doi.org/10.1016/j.gca.2014.04.037
Jones LC, Peters B, Pacheco JS, Lezama, Casciotti KL, Scott F (2015) Stable isotopes and iron oxide mineral products as markers of chemodenitrification. Environ Sci Technol 49(6):3444–3452. https://doi.org/10.1021/es504862x
Kappler A, Pasquero C, Konhauser KO, Newman DK (2005) Deposition of banded iron formations by anoxygenic phototrophic Fe(II)-oxidizing bacteria. Geology 33(11):865–868. https://doi.org/10.1130/G21658.1
Klueglein N, Kappler A (2013) Abiotic oxidation of Fe(II) by reactive nitrogen species in cultures of the nitrate-reducing Fe(II) oxidizer Acidovorax sp. BoFeN1 - questioning the existence of enzymatic Fe(II) oxidation. Geobiology 11(2):180–190. https://doi.org/10.1111/gbi.12019
Klueglein N, Zeitvogel F, Stierhof YD, Floetenmeyer M, Konhauser KO, Kappler A, Obst M (2014) Potential role of nitrite for abiotic Fe(II) oxidation and cell encrustation during nitrate reduction by denitrifying bacteria. Appl Environ Microbiol 80(3):1051–1061. https://doi.org/10.1128/AEM.03277-13
Lack JG, Chaudhuri SK, Chakraborty R, Achenbach LA, Coates JD (2002) Anaerobic biooxidation of Fe(II) by Dechlorosoma suillum. Microb Ecol 43(4):424–431. https://doi.org/10.1007/s00248-001-1061-1
Lalucat J, Bennasar A, Bosch R, García-Valdés E, Palleroni NJ (2006) Biology of Pseudomonas stutzeri. Microbiol Mol Biol Rev 70(2):510–547. https://doi.org/10.1128/MMBR.00047-05
Larese-Casanova P, Haderlein SB, Kappler A (2010) Biomineralization of lepidocrocite and goethite by nitrate-reducing Fe(II)-oxidizing bacteria: effect of pH, bicarbonate, phosphate, and humic acids. Geochim Cosmochim Acta 74(13):3721–3734. https://doi.org/10.1016/j.gca.2010.03.037
Laufer K, Nordhoff M, Røy H, Schmidt C, Behrens S, Jørgensen BB, Kappler A (2015) Coexistence of microaerophilic, nitrate-reducing, and phototrophic Fe(II) oxidizers and Fe(III) reducers in coastal marine sediment. Appl Environ Microbiol 82(5):1433–1447. https://doi.org/10.1128/AEM.03527-15
Li X, Zhang W, Liu T, Chen L, Chen P, Li F (2016) Changes in the composition and diversity of microbial communities during anaerobic nitrate reduction and Fe(II) oxidation at circumneutral pH in paddy soil. Soil Biol Biochem 94:70–79. https://doi.org/10.1016/j.soilbio.2015.11.013
Lovley DR, Phillips EJ (1987) Rapid assay for microbially reducible ferric iron in aquatic sediments. Appl Environ Microbiol 53(7):1536–1540
Mcilvin MR, Altabet MA (2005) Chemical conversion of nitrate and nitrite to nitrous oxide for nitrogen and oxygen isotopic analysis in freshwater and seawater. Anal Chem 77(17):5589–5595. https://doi.org/10.1021/ac050528s
Mejia J, Roden EE, Ginder-vogel MA (2016) Influence of oxygen and nitrate on Fe (hydr)oxide mineral transformation and soil microbial communities during redox cycling. Environ Sci Technol 50(7):3580–3588. https://doi.org/10.1021/acs.est.5b05519
Melton ED, Swanner ED, Behrens S, Schmidt C, Kappler A (2014) The interplay of microbially mediated and abiotic reactions in the biogeochemical Fe cycle. Nat Rev Microbiol 12(12):797–808. https://doi.org/10.1038/nrmicro3347
Miot J, Benzerara K, Morin G, Bernard S, Beyssac O, Larquet E, Kappler A, Guyot F (2009) Transformation of vivianite by anaerobic nitrate-reducing iron-oxidizing bacteria. Geobiology 7(3):373–384. https://doi.org/10.1111/j.1472-4669.2009.00203.x
Muehe EM, Gerhardt S, Schink B, Kappler A (2009) Ecophysiology and the energetic benefit of mixotrophic Fe(II) oxidation by various strains of nitrate-reducing bacteria. FEMS Microbiol Ecol 70(3):335–343. https://doi.org/10.1111/j.1574-6941.2009.00755.x
Nordhoff M, Tominski C, Halama M, Byrne JM, Obst M, Kleindienst S, Behrens S, Kappler A (2017) Insights into nitrate-reducing Fe(II) oxidation mechanisms by analyzing cell-mineral associations, cell encrustation and mineralogy in the chemolithoautotrophic enrichment culture KS. Appl Environ Microbiol 83(13):e00752–e00717. https://doi.org/10.1128/AEM.00752-17
Senko JM, Dewers TA, Krumholz LR (2005) Effect of oxidation rate and Fe(II) state on microbial nitrate-dependent Fe(III) mineral formation. Appl Environ Microbiol 71(11):7172–7177. https://doi.org/10.1128/AEM.71.11.7172-7177.2005
Sørensen J, Thorling L (1991) Stimulation by lepidocrocite (γ-FeOOH) of Fe(II)-dependent nitrite reduction. Geochim Cosmochim Acta 55(5):1289–1294. https://doi.org/10.1016/0016-7037(91)90307-Q
Straub KL, Buchholz-cleven BE (1998) Enumeration and detection of anaerobic ferrous iron-oxidizing, nitrate-reducing bacteria from diverse European sediments. Appl Environ Microbiol 64(12):4846–4856
Straub KL, Benz M, Schink B, Widdel F (1996) Anaerobic, nitrate-dependent microbial oxidation of ferrous iron. Appl Environ Microbiol 62(4):1458–1460
Su JF, Shao SC, Huang TL, Ma F, Yang SF, Zhou ZM, Zheng SC (2015) Anaerobic nitrate-dependent iron(II) oxidation by a novel autotrophic bacterium, Pseudomonas sp. SZF15. J Environ Chem Eng 3(3):2187–2193. https://doi.org/10.1016/j.jece.2015.07.030
Sutka RL, Ostrom NE, Ostrom PH, Breznak JA, Gandhi H, Pitt AJ, Li F (2006) Distinguishing nitrous oxide production from nitrification and denitrification on the basis of isotopomer abundances. Appl Environ Microbiol 72(1):638–644. https://doi.org/10.1128/AEM.72.1.638-644.2006
Tai YL, Dempsey BA (2009) Nitrite reduction with hydrous ferric oxide and Fe(II): stoichiometry, rate, and mechanism. Water Res 43(2):546–552. https://doi.org/10.1016/j.watres.2008.10.055
Toyoda S, Mutobe H, Yamagishi H, Yoshida N, Tanji Y (2005) Fractionation of N2O isotopomers during production by denitrifier. Soil Biol Biochem 37(8):1535–1545. https://doi.org/10.1016/j.soilbio.2005.01.009
Wang M, Hu R, Zhao J, Kuzyakov Y, Liu S (2016) Iron oxidation affects nitrous oxide emissions via donating electrons to denitrification in paddy soils. Geoderma 271:173–180. https://doi.org/10.1016/j.geoderma.2016.02.022
Weber KA, Achenbach LA, Coates JD (2006) Microorganisms pumping iron: anaerobic microbial iron oxidation and reduction. Nat Rev Microbiol 4(10):752–764. https://doi.org/10.1038/nrmicro1490
Wunderlin P, Mohn J, Joss A, Emmenegger L, Siegrist H (2012) Mechanisms of N2O production in biological wastewater treatment under nitrifying and denitrifying conditions. Water Res 46(4):1027–1037. https://doi.org/10.1016/j.watres.2011.11.080
Xu B, Enfors SO (1996) Modeling of nitrite accumulation by the denitrifying bacterium Pseudomonas stutzeri. J Ferment Bioeng 82(1):56–60. https://doi.org/10.1016/0922-338X(96)89455-4
Yu HY, Wang X, Li F, Li B, Liu C, Wang Q, Lei J (2017) Arsenic mobility and bioavailability in paddy soil under iron compound amendments at different growth stages of rice. Environ Pollut 224:136–147. https://doi.org/10.1016/j.envpol.2017.01.072
Zhang J, Zhou W, Liu B, He J, Shen Q, Zhao FJ (2015) Anaerobic arsenite oxidation by an autotrophic arsenite-oxidizing bacterium from an arsenic-contaminated paddy soil. Environ Sci Technol 49(10):5956–5964. https://doi.org/10.1021/es506097c
Zhao L, Dong H, Kukkadapu R, Agrawal A, Liu D, Zhang J, Edelmann RE (2013) Biological oxidation of Fe(II) in reduced nontronite coupled with nitrate reduction by Pseudogulbenkiania sp. strain 2002. Geochim Cosmochim Acta 119:231–247. https://doi.org/10.1016/j.gca.2013.05.033
Zumft WG (1997) Cell biology and molecular basis of denitrification. Microbiol Mol Biol Rev 61(4):533–616
Funding
This work was funded by the National Natural Science Foundations of China (41330857 and 41571130052), the Guangdong Natural Science Funds for Distinguished Young Scholars (2017A030306010), the Australian Research Council DECRA grant (DE150100500) and the SPICC Program (Scientific Platform and Innovation Capability Construction Program of GDAS).
Author information
Authors and Affiliations
Corresponding author
Additional information
Responsible editor: Jizheng He
Electronic supplementary material
ESM 1
(DOC 145 kb)
Rights and permissions
About this article
Cite this article
Li, S., Li, X. & Li, F. Fe(II) oxidation and nitrate reduction by a denitrifying bacterium, Pseudomonas stutzeri LS-2, isolated from paddy soil. J Soils Sediments 18, 1668–1678 (2018). https://doi.org/10.1007/s11368-017-1883-1
Received:
Accepted:
Published:
Issue Date:
DOI: https://doi.org/10.1007/s11368-017-1883-1