Abstract
Aims
Fungi play a central role in litter decomposition, a key process controlling the terrestrial carbon cycle and nutrient availability for plants and microorganisms. Climate change and elevated CO2 affect soil fungi, but the relative importance of the global change variables for litter decomposition is still uncertain. The main objective was therefore to assess the short-term litter decomposition and associated fungal community in a global change manipulated temperate heath ecosystem.
Methods
The heath had been exposed to 6 years of warming, elevated atmospheric CO2 and an extended pre-summer drought. Litterbags with litter from heather (Calluna vulgaris) and wavy-hair grass (Deschampsia flexuosa) were incubated in the litter layer for 6 months, where after we analyzed the litter-associated fungal community, litter loss, CO2 respiration, and total content of carbon, nitrogen and phosphorus.
Results
Elevated temperature tended to increase litter decomposition rates, whereas elevated CO2 had no effect on the process. The pre-summer drought treatment had a positive impact on litter decomposition, CO2 respiration and fungal abundance in the litterbags, although we observed no major changes in fungal community composition.
Conclusions
The drought treatment during pre-summer had a legacy effect on litter decomposition as decomposition rates were positively affected later in the year. The community structure of litter-decomposing fungi was not affected by the drought treatment. Hence, the legacy effect was not mediated by a change in the fungal community structure.



Similar content being viewed by others
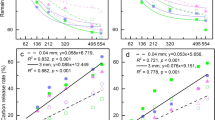
References
Abarenkov K, Nilsson RH, Larsson KH, Alexander IJ, Eberhardt U, Erland S, Høiland K, Kjøller R, Larsson E, Pennanen T, Sen R, Taylor AFS, Tedersoo L, Ursing BM, Vrålstad T, Liimatainen K, Peintner U, Kõljalg U (2010) The UNITE database for molecular identification of fungi - recent updates and future perspectives. New Phytol 186:281–285
Aerts R (1997) Climate, leaf litter chemistry and leaf litter decomposition in terrestrial ecosystems: a triangular relationship. Oikos 79:439–449
Allison SD, Treseder KK (2008) Warming and drying suppress microbial activity and carbon cycling in boreal forest soils. Glob Chang Biol 14:2898–2909
Anderson IC, Drigo B, Keniry K, Ghannoum O, Chambers SM, Tissue DT, Cairney JWG (2013) Interactive effects of preindustrial, current and future atmospheric CO2 concentrations and temperature on soil fungi associated with two Eucalyptus species. FEMS Microbiol Ecol 83:425–437
Andresen LC, Michelsen A, Jonasson S, Schmidt IK, Mikkelsen TN, Ambus P, Beier C (2010) Plant nutrient mobilization in temperate heathland responds to elevated CO2, temperature and drought. Plant Soil 328:381–396
Andresen LC, Konestabo HS, Maraldo K, Holmstrup M, Ambus P, Beier C, Michelsen A (2011) Organic matter flow in the food web at a temperate heath under multifactorial climate change. Rapid Commun Mass Spectrom 25:1485–1496
Barnard RL, Osborne CA, Firestone MK (2013) Responses of soil bacterial and fungal communities to extreme desiccation and rewetting. ISME J 7:2229–2241
Bontti EE, Decant JP, Munson SM, Gathany MA, Przeszlowska A, Haddix ML, Owens S, Burke IC, Parton WJ, Harmon M (2009) Litter decomposition in grasslands of Central North America (US Great Plains). Glob Chang Biol 15:1356–1363
Butenschoen O, Scheu S, Eisenhauer N (2011) Interactive effects of warming, soil humidity and plant diversity on litter decomposition and microbial activity. Soil Biol Biochem 43:1902–1907
Christensen JH, Christensen OB (2007) A summary of the PRUDENCE model projections of changes in European climate by the end of this century. Clim Chang 81:7–30
Cotrufo MF, Ineson P, Scott A (1998) Elevated CO2 reduces the nitrogen concentration of plant tissues. Glob Chang Biol 4:43–54
Couteaux MM, Bottner P, Berg B (1995) Litter decomposition, climate and litter quality. Trends Ecol Evol 10:63–66
de Boer W, Folman LB, Summerbell RC, Boddy L (2005) Living in a fungal world: impact of fungi on soil bacterial niche development. FEMS Microbiol Rev 29:795–811
de Vries FT, Liiri ME, Bjørnlund L, Bowker MA, Christensen S, Setälä HM, Bardgett RD (2012) Land use alters the resistance and resilience of soil food webs to drought. Nat Clim Chang 2:276–280
Drigo B, Kowalchuk GA, van Veen JA (2008) Climate change goes underground: effects of elevated atmospheric CO2 on microbial community structure and activities in the rhizosphere. Biol Fertil Soils 44:667–679
Emmett BA, Beier C, Estiarte M, Tietema A, Kristensen HL, Williams D, Peñuelas J, Schmidt I, Sowerby A (2004) The response of soil processes to climate change: results from manipulation studies of shrublands across an environmental gradient. Ecosystems 7:625–637
Faber JH (1991) Functional classification of soil fauna: a new approach. Oikos 62:110–117
Fierer N, Jackson JA, Vilgalys R, Jackson RB (2005) Assessment of soil microbial community structure by use of taxon-specific quantitative PCR assays. Appl Environ Microbiol 71:4117–4120
Frankland JC (1998) Fungal succession — unravelling the unpredictable. Mycol Res 102:1–15
Frey SD, Elliott ET, Paustian K (1999) Bacterial and fungal abundance and biomass in conventional and no-tillage agroecosystems along two climatic gradients. Soil Biol Biochem 31:573–585
Gonzalez P, Neilson RP, Lenihan JM, Drapek RJ (2010) Global patterns in the vulnerability of ecosystems to vegetation shifts due to climate change. Glob Ecol Biogeogr 19:755–768
Harmon ME, Nadelhoffer KJ, Blair JM (1999) Measuring decomposition, nutrient turnover, and stores in plant litter. In: Robertson GP, Coleman DC, Bledsoe CS, Sollins P (eds) Standard soil methods for long-term ecological research. Oxford University Press, Oxford, United Kingdom, pp. 202–240
Haugwitz MS, Bergmark L, Priemé A, Christensen S, Beier C, Michelsen A (2014) Soil microorganisms respond to five years of climate change manipulations and elevated atmospheric CO2 in a temperate heath ecosystem. Plant Soil 374:211–222
Holmstrup M, Sørensen JG, Schmidt IK, Nielsen PL, Masonc S, Tietemac A, Smith AR, Bataillon T, Beier C, Ehlers BK (2013) Soil microarthropods are only weakly impacted after 13 years of repeated drought treatment in wet and dry heathland soils. Soil Biol Biochem 66:110–118
Ihrmark K, Bödeker ITM, Cruz-Martinez K, Friberg H, Kubartova A, Schenck J, Strid Y, Stenlid J, Brandström-Durling M, Clemmensen KE, Lindahl BD (2012) New primers to amplify the fungal ITS2 region – evaluation by 454-sequencing of artificial and natural communities. FEMS Microbiol Ecol 82:666–677
Incerti G, Bonanomi G, Giannino F, Rutigliano FA, Piermatteo D, Castaldi S, De Marco A, Fierro A, Fioretto A, Maggi O, Papa S, Persiani AM, Feoli E, De Santo AV, Mazzoleni S (2011) Litter decomposition in Mediterranean ecosystems: Modelling the controlling role of climatic conditions and litter quality. Appl Soil Ecol 49:148–157
IPCC (2007) Climate change 2007: the physical science basis. In: Solomon S, Qin D, Manning M, et al. (eds) Climate change 2007: impacts, adaptation and vulnerability. Contribution of Working Group II to the Fourth Assessment Report of the Intergovernmental Panel on Climate Change. Cambridge University Press, Cambridge, United Kingdom
Jensen KD, Beier C, Michelsen A, Emmett BA (2003) Effects of experimental drought on microbial processes in two temperate heathlands at contrasting water conditions. Appl Soil Ecol 24:165–176
Jonasson S, Michelsen A, Schmidt IK, Nielsen EV, Callaghan TV (1996) Microbial biomass C, N and P in two arctic soils and responses to addition of NPK fertilizer and sugar: implications for plant nutrient uptake. Oecologia 106:507–515
Khalvati MA, Hu Y, Mozafar A, Schmidhalter U (2005) Quantification of water uptake by arbuscular mycorrhizal hyphae and its significance for leaf growth, water relations, and gas exchange of barley subjected to drought stress. Plant Biol (Stuttgart) 7:706–712
Kjøller A, Struwe S (1982) Microfungi in ecosystems - fungal occurrence and activity in litter and soil. Oikos 39:391–422
Liu LL, King JS, Giardina CP, Booker FL (2009) The influence of chemistry, production and community composition on leaf litter decomposition under elevated atmospheric CO2 and tropospheric O3 in a northern hardwood ecosystem. Ecosystems 12:401–416
Manzoni S, Schimel JP, Porporato A (2012) Responses of microbial communties to water stress: results from a meta-analysis. Ecology 93:930–938
Maraldo K, Holmstrup M (2009) Recovery of enchytraeid populations after severe drought events. Appl Soil Ecol 42:227–235
Maraldo K, Krogh PH, van der Linden L, Christensen B, Mikkelsen TN, Beier C, Holmstrup M (2010) The counteracting effects of elevated atmospheric CO2 concentrations and drought episodes: studies of enchytraeid communities in a dry heathland. Soil Biol Biochem 42:1958–1966
Mikkelsen TN, Beier C, Jonasson S, et al. (2008) Experimental design of multifactor climate change experiments with elevated CO2, warming and drought: the CLIMAITE project. Funct Ecol 22:185–195
Nielsen PL, Andresen LC, Michelsen A, Schmidt IK, Kongstad J (2009) Seasonal variations and effects of nutrient applications on N and P and microbial biomass under two temperate heathland plants. Appl Soil Ecol 42:279–287
Norby RJ, Cotrufo MF (1998) Global change - a question of litter quality. Nature 396:17–18
Norby RJ, Cotrufo MF, Ineson P, O'Neill EG, Canadell JG (2001) Elevated CO2, litter chemistry, and decomposition: a synthesis. Oecologia 127:153–165
Osono T, Takeda H (2002) Comparison of litter decomposing ability among diverse fungi in a cool temperate deciduous forest in Japan. Mycologia 94:421–427
Penuelas J, Gordon C, Llorens L, Nielsen T, Tietema A, Beier C, Bruna P, Emmett B, Estiarte M, Gorissen A (2004) Nonintrusive field experiments show different plant responses to warming and drought among sites, seasons, and species in a north-south European gradient. Ecosystems 7:598–612
Robinson CL, Dighton J, Frankland J (1993) Resource capture by interacting fungal colonizers of straw. Mycol Res 97:547–558
Sanaullah M, Rumpel C, Charrier X, Chabbi A (2012) How does drought stress influence the decomposition of plant litter with contrasting quality in a grassland ecosystem? Plant Soil 352:277–288
Seastedt TR (1984) The role of microarthropods in decomposition and mineralization processes. Annu Rev Entomol 29:25–46
Selsted MB, van der Linden L, Ibrom A, Michelsen A, Larsen KS, Pedersen JK, Mikkelsen TN, Pilegaard K, Beier C, Ambus P (2012) Soil respiration is stimulated by elevated CO2 and reduced by summer drought: three years of measurements in a multifactor ecosystem manipulation experiment in a temperate heathland (CLIMAITE). Glob Chang Biol 18:1216–1230
Toberman H, Evans CD, Freeman C, Fenner N, Artz RRE (2008) Summer drought effects upon soil and litter extracellular phenol oxidase activity and soluble carbon release in an upland Calluna heathland. Soil Biol Biochem 40:1519–1532
Trenberth KE, Dai A, van der Schrier G, Jones PD, Barichivich J, Briffa KR, Sheffield J (2014) Global warming and changes in drought. Nat Clim Chang 4:17–22
Unterseher M, Jumpponen A, Opik M, Tedersoo L, Moora M, Dormann CF, Schnittler M (2011) Species abundance distributions and richness estimations in fungal metagenomics - lessons learned from community ecology. Mol Ecol 20:275–285
van Meeteren MM, Tietema A, van Loon EE, Verstraten JM (2008) Microbial dynamics and litter decomposition under a changed climate in a Dutch heathland. Appl Soil Ecol 38:119–127
White TJ, Bruns TD, Lee SB, Taylor JW (1990) Amplification and direct sequencing of fungal ribosomal RNA genes for phylogenetics. In: Gelfand DH, Sninsky JJ, White TJ (eds) Innis MA. PCR Protocols - a Guide to Methods and Applications. Academic Press, San Diego, CA, USA, pp. 315–322
Yu ZT, Mohn WW (1999) Killing two birds with one stone: simultaneous extraction of DNA and RNA from activated sludge biomass. Can J Microbiol 45:269–272
Yuste JC, Penuelas J, Estiarte M, Garcia-Mas J, Mattana S, Ogaya R, Pujol M, Sardans J (2011) Drought-resistant fungi control soil organic matter decomposition and its response to temperature. Glob Chang Biol 17:1475–1486
Zhang W, Parker KM, Luo Y, Wan S, Wallace LL, Hu S (2005) Soil microbial responses to experimental warming and clipping in a tallgrass prairie. Glob Chang Biol 11:266–277
Acknowledgments
This study was carried out within the CLIMAITE project, financially supported by the Villum Kann Rasmussen Foundation. We thank Karin Vestberg, Esben V. Nielsen and Anette Hørdum Løth for excellent technical assistance.
Author information
Authors and Affiliations
Corresponding author
Additional information
Responsible Editor: Duncan D. Cameron.
Electronic supplementary material
ESM 1
(DOCX 15 kb)
ESM 2
Fig. S1 The CLIMAITE experimental site; a) A schematic of the global change treatments with each block consisting of two octagons: one with ambient CO2 and one with elevated CO2. Each octagon includes the treatments drought (−H2O), warming (+T) and the combination plus either an ambient “control plot” or an elevated CO2 plot. In total, there are 12 octagons at the experimental site giving 48 plots. b) Picture of the vegetation density at the CLIMAITE experimental site in October 2011. The dominant plant species are Calluna vulgaris and Deschampsia flexuoxa. (PNG 21290 kb)
ESM 3
Fig. S2 Weekly averages of climatic data from 2011 at the CLIMAITE experimental site; a) Soil water content (vol.% in 0–20 cm soil depth) in plots without pre-summer drought treatment (filled triangles) and plots with pre-summer drought treatment (open triangles). Daily precipitation (mm) is shown in light-grey bars; b) Soil surface temperature in plots without temperature treatment (filled circles) and plots with temperature treatment (open circles). (GIF 64 kb)
ESM 4
Fig. S3 The relationship between litter loss (%) and fungal ITS copy number (g−1 soil) after six months of incubation of litterbags in the global change experiment CLIMIATE. Each filled circle represents one sample (n = 48). (GIF 15 kb)
Rights and permissions
About this article
Cite this article
Haugwitz, M.S., Michelsen, A. & Priemé, A. The legacy of climate change effects: previous drought increases short-term litter decomposition rates in a temperate mixed grass- and shrubland. Plant Soil 408, 183–193 (2016). https://doi.org/10.1007/s11104-016-2913-2
Received:
Accepted:
Published:
Issue Date:
DOI: https://doi.org/10.1007/s11104-016-2913-2