Abstract
Thermoresponsive shape memory poly(ε-caprolactone) (PCL) networks with readily tunable properties are prepared exploiting the facile UV-initiated thiol–ene methodology. PCLs with multiple pendant double bonds at both chain ends are used to cross-link with multi-thiol compounds via photo-initiated thiol–ene reactions. Mechanical and shape memory properties can be altered through changes in the number of alkene side groups at the PCL chain termini and thiol cross-linker structure. In parallel with structural investigation by spectroscopic techniques, the relationships between the composition and material properties are investigated. The PCL thermoset materials exhibit excellent thermoresponsive shape memory performance by changing the operation temperature below or above the melting temperature of crystalline PCL segments, which varies in the range of 34–40 °C, and the highest shape retention and recovery ratios can reach 98–100%.











Similar content being viewed by others
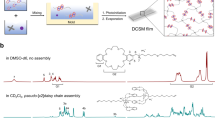
References
Behl M, Lendlein A (2007) Shape-memory polymers. Mater Today 10:20–28
Zhao Q, Qi HJ, Xie T (2015) Recent progress in shape memory polymer: new behavior, enabling materials, and mechanistic understanding. Prog Polym Sci 49–50:79–120
Hager MD, Bode S, Weber C, Schubert US (2015) Shape memory polymers: past, present and future developments. Prog Polym Sci 49–50:3–33
Liu C, Qin H, Mather PT (2007) Review of progress in shape-memory polymers. J Mater Chem 17:1543–1558
Pilate F, Toncheva A, Dubois P, Raquez J-M (2016) Shape-memory polymers for multiple applications in the materials world. Eur Polym J 80:268–294
Lendlein A, Sauter T (2013) Shape-memory effect in polymers. Macromol Chem Phys 214:1175–1177
Behl M, Razzaq MY, Lendlein A (2010) Multifunctional shape-memory polymers. Adv Mater 22:3388–3410
Woodruff MA, Hutmacher DW (2010) The return of a forgotten polymer: polycaprolactone in the 21st century. Prog Polym Sci 35:1217–1256
Rainbolt EA, Washington KE, Biewer MC, Stefan MC (2015) Recent developments in micellar drug carriers featuring substituted poly(ε-caprolactone)s. Polym Chem 6:2369–2381
Salvekar AV, Zhou Y, Huang WM et al (2015) Shape/temperature memory phenomena in un-crosslinked poly-ɛ-caprolactone (PCL). Eur Polym J 72:282–295
Lendlein A, Langer R (2002) Biodegradable, elastic shape-memory polymers for potential biomedical applications. Science 296:1673–1676
Lutz A, van den Berg O, Van Damme J et al (2014) A shape-recovery polymer coating for the corrosion protection of metallic surfaces. ACS Appl Mater Interfaces 7:175–183
Jorcin J-B, Scheltjens G, Van Ingelgem Y et al (2010) Investigation of the self-healing properties of shape memory polyurethane coatings with the ‘odd random phase multisine’ electrochemical impedance spectroscopy. Electrochim Acta 55:6195–6203
González-García Y, Mol JMC, Muselle T et al (2011) A combined mechanical, microscopic and local electrochemical evaluation of self-healing properties of shape-memory polyurethane coatings. Electrochim Acta 56:9619–9626
Nagahama K, Ueda Y, Ouchi T, Ohya Y (2009) Biodegradable shape-memory polymers exhibiting sharp thermal transitions and controlled drug release. Biomacromolecules 10:1789–1794
Rivero G, Nguyen L-TT, Hillewaere XKD, Du Prez FE (2014) One-pot thermo-remendable shape memory polyurethanes. Macromolecules 47:2010–2018
Nguyen L-TT, Truong TT, Nguyen HT et al (2015) Healable shape memory (thio)urethane thermosets. Polym Chem 6:3143–3154
Nguyen L-TT, Nguyen HT, Truong TT (2015) Thermally mendable material based on a furyl-telechelic semicrystalline polymer and a maleimide crosslinker. J Polym Res 22:186
Lendlein A, Behl M, Hiebl B, Wischke C (2010) Shape-memory polymers as a technology platform for biomedical applications. Expert Rev Med Devices 7:357–379
D’hollander S, Assche GV, Mele BV, Du Prez F (2009) Novel synthetic strategy toward shape memory polyurethanes with a well-defined switching temperature. Polymer 50:4447–4454
Momtaz M, Razavi-Nouri M, Barikani M (2014) Effect of block ratio and strain amplitude on thermal, structural, and shape memory properties of segmented polycaprolactone-based polyurethanes. J Mater Sci 49:7575–7584. doi:10.1007/s10853-014-8466-y.pdf
Peponi L, Navarro-Baena I, Sonseca A, Gimenez E, Marcos-Fernandez A, Kenny JM (2013) Synthesis and characterization of PCL–PLLA polyurethane with shape memory behavior. Eur Polym J 49:893–903
Rana S, Cho JW, Tan LP (2013) Graphene-crosslinked polyurethane block copolymer nanocomposites with enhanced mechanical, electrical, and shape memory properties. RSC Adv 3:13796–13803
Berg GJ, McBride MK, Wang C, Bowman CN (2014) New directions in the chemistry of shape memory polymers. Polymer 55:5849–5872
Ping P, Wang W, Chen X, Jing X (2005) Poly(ε-caprolactone) polyurethane and its shape-memory property. Biomacromolecules 6:587–592
Wu X, Liu L, Fang W, Qiao C, Li T (2016) Effect of hard segment architecture on shape memory properties of polycaprolactone-based polyurethane containing azobenzene. J Mater Sci 51:2727–2738. doi:10.1007/s10853-015-9586-8
Zotzmann J, Behl M, Feng Y, Lendlein A (2010) Copolymer networks based on poly(ω-pentadecalactone) and poly(ϵ-caprolactone)segments as a versatile triple-shape polymer system. Adv Funct Mater 20:3583–3594
Xue L, Dai S, Li Z (2010) Biodegradable shape-memory block co-polymers for fast self-expandable stents. Biomaterials 31:8132–8140
Zhu G, Xu S, Wang J, Zhang L (2006) Shape memory behaviour of radiation-crosslinked PCL/PMVS blends. Radiat Phys Chem 75:443–448
Zhu GM, Xu QY, Liang GZ, Zhou HF (2005) Shape-memory behaviors of sensitizing radiation-crosslinked polycaprolactone with polyfunctional poly(ester acrylate). J Appl Polym Sci 95:634–639
Zhu G, Liang G, Xu Q, Yu Q (2003) Shape-memory effects of radiation crosslinked poly(ϵ-caprolactone). J Appl Polym Sci 90:1589–1595
Nagata M, Yamamoto Y (2009) Synthesis and characterization of photocrosslinked poly(ε-caprolactone)s showing shape-memory properties. J Polym Sci A Polym Chem 47:2422–2433
Nagata M, Sato Y (2005) Synthesis and properties of photocurable biodegradable multiblock copolymers based on poly(ε-caprolactone) and poly(L-lactide) segments. J Polym Sci A Polym Chem 43:2426–2439
Nagata M, Kitazima I (2006) Photocurable biodegradable poly(ɛ-caprolactone)/poly(ethylene glycol) multiblock copolymers showing shape-memory properties. Colloid Polym Sci 284:380–386
Nagata M, Yamamoto Y (2010) Photocurable shape-memory copolymers of ε-caprolactone and L-lactide. Macromol Chem Phys 211:1826–1835
Neffe AT, Hanh BD, Steuer S, Lendlein A (2009) Polymer networks combining controlled drug release, biodegradation, and shape memory capability. Adv Mater 21:3394–3398
Lendlein A, Schmidt AM, Schroeter M, Langer R (2005) Shape-memory polymer networks from oligo(ϵ-caprolactone)dimethacrylates. J Polym Sci A Polym Chem 43:1369–1381
Lendlein A, Schmidt AM, Langer R (2001) AB-polymer networks based on oligo(ɛ-caprolactone) segments showing shape-memory properties. Proc Natl Acad Sci USA 98:842–847
Schmidt AM (2006) Electromagnetic activation of shape memory polymer networks containing magnetic nanoparticles. Macromol Rapid Commun 27:1168–1172
Zhou J, Schmidt AM, Ritter H (2010) Bicomponent transparent polyester networks with shape memory effect. Macromolecules 43:939–942
Garle A, Kong S, Ojha U, Budhlall BM (2012) Thermoresponsive semicrystalline poly(ε-caprolactone) networks: exploiting cross-linking with cinnamoyl moieties to design polymers with tunable shape memory. ACS Appl Mater Interfaces 4:645–657
Paderni K, Pandini S, Passera S, Pilati F, Toselli M, Messori M (2012) Shape-memory polymer networks from sol–gel cross-linked alkoxysilane-terminated poly(ε-caprolactone). J Mater Sci 47:4354–4362. doi:10.3390/ma7020751
Defize T, Riva RR, Raquez J-M, Dubois P, Jérôme C, Alexandre M (2011) Thermoreversibly crosslinked poly(ε-caprolactone) as recyclable shape-memory polymer network. Macromol Rapid Commun 32:1264–1269
Defize T, Thomassin J-M, Alexandre M, Gilbert B, Riva R, Jérôme C (2016) Comprehensive study of the thermo-reversibility of Diels-Alder based PCL polymer networks. Polymer 84:234–242
Boire TC, Gupta MK, Zachman AL et al (2015) Pendant allyl crosslinking as a tunable shape memory actuator for vascular applications. Acta Biomater 24:53–63
Basko M, Bednarek M, Vlaminck L, Kubisa P, Du Prez FE (2017) Biodegradable polymer networks via triazolinedione-crosslinking of oleyl-functionalized poly(ε-caprolactone). Eur Polym J 89:230–240
Hoyle CE, Bowman CN (2010) Thiol–ene Click Chemistry. Angew Chem Int Ed 49:1540–1573
Lowe AB (2010) Thiol–ene “click” reactions and recent applications in polymer and materials synthesis. Polym Chem 1:17–36
Kade MJ, Burke DJ, Hawker CJ (2010) The power of thiol–ene chemistry. J Polym Sci A Polym Chem 48:743–750
Yang G, Kristufek SL, Link LA, Wooley KL, Robertson ML (2015) Synthesis and physical properties of thiol–ene networks utilizing plant-derived phenolic acids. Macromolecules 48:8418–8427
Nguyen L-TT, Gokmen MT, Du Prez FE (2013) Kinetic comparison of 13 homogeneous thiol-X reactions. Polym Chem 4:5527–5536
Lu H, Carioscia JA, Stansbury JW, Bowman CN (2005) Investigations of step-growth thiol–ene polymerizations for novel dental restoratives. Dent Mater 21:1129–1136
Machado TO, Sayer C, Araujo PHH (2017) Thiol–ene polymerisation: a promising technique to obtain novel biomaterials. Eur Polym J 86:200–215
Lee KM, Knight PT, Chung T, Mather PT (2008) Polycaprolactone–POSS chemical/physical double networks. Macromolecules 41:4730–4738
Rodriguez ED, Luo X, Mather PT (2011) Linear/network poly(ε-caprolactone) blends exhibiting shape memory assisted self-healing (SMASH). ACS Appl Mater Interfaces 3:152–161
Baker RM, Henderson JH, Mather PT (2013) Shape memory poly(ε-caprolactone)-co-poly(ethylene glycol) foams with body temperature triggering and two-way actuation. J Mater Chem B 1:4916–4920
Alvarado-Tenorio B, Romo-Uribe A, Mather PT (2011) Microstructure and phase behavior of POSS/PCL shape memory nanocomposites. Macromolecules 44:5682–5692
Nottelet B, Tambutet G, Bakkour Y, Coudane J (2012) Redox and thiol–ene cross-linking of mercapto poly(ε-caprolactone) for the preparation of reversible degradable elastomeric materials. Polym Chem 3:2956–2963
Basko M, Bednarek M, Nguyen L-TT, Kubisa P, Du Prez F (2013) Functionalization of polyurethanes by incorporation of alkyne side-groups to oligodiols and subsequent thiolyne post-modification. Eur Polym J 49:3573–3581
Lin XK, Chen L, Zhao YP, Dong ZZ (2010) Synthesis and characterization of thermoresponsive shape-memory poly(stearyl acrylate-co-acrylamide) hydrogels. J Mater Sci 45:2703–2707. doi:10.1007/s10853-010-4255-4.pdf
Lendlein A, Kelch S (2002) Shape-memory polymers. Angew Chem Int Ed 41:2034–2057
Liu Y, Han C, Tan H, Du X (2010) Thermal, mechanical and shape memory properties of shape memory epoxy resin. Mater Sci Eng A 527:2510–2514
Hearon K, Gall K, Ware T, Maitland DJ, Bearinger JP, Wilson TS (2011) Post-polymerization crosslinked polyurethane shape memory polymers. J Appl Polym Sci 121:144–153
Hoyle CE, Lee TY, Roper T (2004) Thiol–enes: chemistry of the past with promise for the future. J Polym Sci A Polym Chem 42:5301–5338
Coates J (2000) In: Meyers RA (ed) Encyclopedia of analytical chemistry. Wiley, Chichester
Nguyen L-TT, Devroede J, Plasschaert K, Jonckheere L, Haucourt N, Du Prez FE (2013) Providing polyurethane foams with functionality: a kinetic comparison of different “click” and coupling reaction pathways. Polym Chem 4:1546–1556
Derboven P, D’hooge DR, Stamenovic MM et al (2013) Kinetic modeling of radical thiol–ene chemistry for macromolecular design: importance of side reactions and diffusional limitations. Macromolecules 46:1732–1742
Koo SPS, Stamenovi´c MM, Prasath RA et al (2010) Limitations of radical thiol–ene reactions for polymer–polymer conjugation. J Polym Sci A Polym Chem 48:1699–1713
Billiet L, Gok O, Dove AP, Sanyal A, Nguyen L-TT, Du Prez FE (2011) Metal-free functionalization of linear polyurethanes by thiol–maleimide coupling reactions. Macromolecules 44:7874–7878
Rydholm AE, Reddy SK, Anseth KS, Bowman CN (2007) Development and characterization of degradable thiol-allyl ether photopolymers. Polymer 48:4589–4600
Crescenzi V, Manzini G, Calzolari G, Borri C (1972) Thermodynamics of fusion of poly-β-propiolactone and poly-ϵ-caprolactone. comparative analysis of the melting of aliphatic polylactone and polyester chains. Eur Polym J 8:449–463
Hu J, Zhu Y, Huang H, Lu J (2012) Recent advances in shape–memory polymers: structure, mechanism, functionality, modeling and applications. Prog Polym Sci 37:1720–1763
Xie T (2011) Recent advances in polymer shape memory. Polymer 52:4985–5000
Acknowledgements
This research was fully supported by Vietnam National Foundation for Science and Technology Development (NAFOSTED) under Grant Number “104.02-2015.95.” We acknowledge Vinh Truong, Tri M. Phan and Viet Q. Nguyen for their assistance to the experiments and analysis. Coenraad Schaap (Perstorp AB) and Malin Falkman (Perstorp AB) are acknowledged for advice and for supplying the polycaprolactone product. Elvira Schlatter (Bruno Bock) and Matthias Rehfeld (Bruno Bock) are acknowledged for advice and for kindly providing the tetra-functional thiol products.
Author information
Authors and Affiliations
Corresponding author
Ethics declarations
Conflict of interest
The authors declare that they have no competing interest.
Electronic supplementary material
Below is the link to the electronic supplementary material.
Rights and permissions
About this article
Cite this article
Truong, T.T., Thai, S.H., Nguyen, H.T. et al. Poly(ε-caprolactone) networks with tunable thermoresponsive shape memory via a facile photo-initiated thiol–ene pathway. J Mater Sci 53, 2236–2252 (2018). https://doi.org/10.1007/s10853-017-1643-z
Received:
Accepted:
Published:
Issue Date:
DOI: https://doi.org/10.1007/s10853-017-1643-z