Abstract
This paper presents an analysis of the combined electro-osmotic and pressure-driven axial flows of viscoelastic fluids in a rectangular microchannel with arbitrary aspect ratios. The rheological behavior of the fluid is described by the complete form of Phan-Thien–Tanner (PTT) model with the Gordon–Schowalter convected derivative which covers the upper convected Maxwell, Johnson–Segalman and FENE-P models. Our numerical simulation is based on the computation of 2D Poisson–Boltzmann, Cauchy momentum and PTT constitutive equations. The solution of these governing nonlinear coupled set of equations is obtained by using the second-order central finite difference method in a non-uniform grid system and is verified against 1D analytical solution of the velocity profile with less than 0.06% relative error. Also, a parametric study is carried out to investigate the effect of channel aspect ratio (width to height), wall zeta potential and the Debye–Hückel parameter on 2D velocity profile, volumetric flow rate and the Poiseuille number in the mixed EO/PD flows of viscoelastic fluids with different Weissenberg numbers. Our results show that, for low channel aspect ratios, the previous 1D analytical models underestimate the velocity profile at the channel half-width centerline in the case of favorable pressure gradients and overestimate it in the case of adverse pressure gradients. The results reveal that the inapplicability of the Debye–Hückel approximation at high zeta potentials is more significant for higher Weissenberg number fluids. Also, it is found that, under the specified values of electrokinetic parameters, there is a threshold for velocity scale ratio in which the Poiseuille number is approximately independent of channel aspect ratio.
Similar content being viewed by others
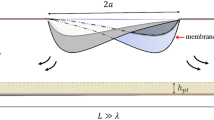
Abbreviations
- APG/FPG:
-
Adverse/favorable pressure gradient
- B, D :
-
Coefficient functions
- \(\mathbf{D}\) :
-
Rate of deformation tensor (\(s^{-1})\)
- e :
-
Elementary charge (\(1.6022\times 10^{-19}C)\)
- \({\varvec{E}},E_z \) :
-
Vector/z-component of electric field (\(Vm^{-1})\)
- \(f\left( {\tau _{kk} } \right) \) :
-
PTT stress coefficient function
- \(f_r \) :
-
Friction factor
- \(k_B \) :
-
Boltzmann constant (\(1.3807\times 10^{-23}JK^{-1})\)
- L, 2H, 2W :
-
Microchannel length/height/width (m)
- M :
-
Coefficients of transformation function
- \(n_0 \) :
-
Ionic number concentration (\(m^{-3})\)
- p :
-
Pressure (Pa)
- Q :
-
Volumetric flow rate \((m^3 s^{-1})\)
- \(\hbox {Re}\) :
-
Reynolds number
- t :
-
Time (s)
- \(T_m \) :
-
Average absolute temperature (K)
- \({\varvec{u}},u\) :
-
Vector/z-component of velocity (\(ms^{-1})\)
- \(\hbox {We}_\kappa \) :
-
Weissenberg number
- x, y, z :
-
Transverse/depth-wise/axial coordinate (m)
- \(\mathbb {z}^{\pm }\) :
-
Valence of ions
- \(\alpha \) :
-
Channel aspect ratio
- \(\beta \) :
-
Stretch parameter
- \(\varGamma \) :
-
Ratio of PD to HS velocities
- \(\Delta \) :
-
Error index
- \(\varepsilon \) :
-
Extensibility parameter
- \(\epsilon \) :
-
Permittivity of the fluid (\(CV^{-1}m^{-1})\)
- \(\phi / \varPhi \) :
-
External/total electrical potential (V)
- \(\eta _p \) :
-
Polymer viscosity coefficient (Pa.s)
- \(\kappa \) :
-
Debye–Hückel parameter (\(m^{-1})\)
- K :
-
Dimensionless Debye–Hückel parameter
- \(\lambda \) :
-
Relaxation time (s)
- \(\xi \) :
-
PTT model parameter
- \(\Pi \Pi \) :
-
Physical domain of the problem (\(m^{2})\)
- \(\rho _e \) :
-
Electric charge density (\(Cm^{-3})\)
- \({\varvec{\tau }} ,\tau _{kk} \) :
-
Polymeric/trace of extra-stress tensor (Pa)
- \(\tau _{xz} ,\tau _{yz} \) :
-
Stream-wise shear stresses (Pa)
- \(\tau _{xx} ,\tau _{yy} \) :
-
Transverse normal stresses (Pa)
- \(\tau _{xy} \) :
-
Transverse shear stress (Pa)
- \(\tau _w \) :
-
Shear stress at the wall (Pa)
- \(\tau _{zz} \) :
-
Stream-wise normal stress (Pa)
- \(\psi ,\psi _0 \) :
-
EDL/wall zeta potential (V)
- \(\omega \) :
-
Parameter to calculate the relative error
- i, j, k :
-
Refer to transverse/depth-wise/axial direction
- HS :
-
Refers to Helmholtz–Smoluchowski
- m :
-
Refers to average of parameter
- \(P,\textit{NB}\) :
-
Refer to central node and neighbor grid point
- PD :
-
Refers to pressure driven
- PTT :
-
Refers to Phan-Thien–Tanner model
- sPTT :
-
Refers to simplified PTT model
- \(\psi ,u\) :
-
Refer to potential and velocity
- g :
-
Relevant to the initial guess
- \(\hbox {T}\) :
-
Transpose of the matrix
- −:
-
Relevant to dimensionless variable/domain
- \({\wedge }\) :
-
Relevant to transformed variable/domain
- \(\square \) :
-
Gordon–Schowalter convected derivative
References
Auroux, P.-A., Iossifidis, D., Reyes, D.R., Manz, A.: Micro total analysis systems. 2. Analytical standard operations and applications. Anal. Chem. 74(12), 2637–2652 (2002)
Reyes, D.R., Iossifidis, D., Auroux, P.-A., Manz, A.: Micro total analysis systems. 1. Introduction, theory, and technology. Anal. Chem. 74(12), 2623–2636 (2002)
Whitesides, G.M.: The origins and the future of microfluidics. Nature 442(7101), 368–373 (2006)
Stone, H.A., Stroock, A.D., Ajdari, A.: Engineering flows in small devices: microfluidics toward a lab-on-a-chip. Annu. Rev. Fluid Mech. 36, 381–411 (2004)
Li, D.: Electrokinetics in microfluidics, vol. 2. Academic Press, Cambridge (2004)
Karniadakis, G., Beskok, A., Aluru, N.R.: Microflows and nanoflows: fundamentals and simulation, vol. 29. Springer, Berlin (2006)
Li, H., Wong, T.N., Nguyen, N.-T., Chai, J.C.: Numerical modeling of tunable optofluidics lens based on combined effect of hydrodynamics and electroosmosis. Int. J. Heat Mass Trans. 55(9), 2647–2655 (2012)
Dutta, P., Beskok, A.: Analytical solution of combined electroosmotic/pressure driven flows in two-dimensional straight channels: finite Debye layer effects. Anal. Chem. 73(9), 1979–1986 (2001)
Horiuchi, K., Dutta, P.: Heat transfer characteristics of mixed electroosmotic and pressure driven micro-flows. JSME Int. J. B-Fluid T. 49(3), 812–819 (2006)
Ren, L., Escobedo, C., Li, D.: Electroosmotic flow in a microcapillary with one solution displacing another solution. J. Colloid Interface Sci. 242(1), 264–271 (2001)
Li, H., Wong, T.N., Nguyen, N.-T.: A tunable optofluidic lens based on combined effect of hydrodynamics and electroosmosis. Microfluid. Nanofluid. 10(5), 1033–1043 (2011)
Lettieri, G.-L., Dodge, A., Boer, G., de Rooij, N.F., Verpoorte, E.: A novel microfluidic concept for bioanalysis using freely moving beads trapped in recirculating flows. Lab Chip 3(1), 34–39 (2003)
Boer, G., Dodge, A., Fluri, K., van der Schoot, B., Verpoorte, E., de Rooij, N.: Studies of hydrostatic pressure effects in electrokinetically driven \(\mu \)TAS. In: Harrison, D.J., van den Berg, A. (eds.) Micro Total Analysis Systems’ 98, Workshop, held in Banff, Canada, 13–16 October 1998, pp. 53–56. Springer, Netherlands, Dordrecht (1998)
Wu, W.-T., Yang, F., Antaki, J.F., Aubry, N., Massoudi, M.: Study of blood flow in several benchmark micro-channels using a two-fluid approach. Int. J. Eng. Sci. 95, 49–59 (2015)
Helton, K.L., Nelson, K.E., Fu, E., Yager, P.: Conditioning saliva for use in a microfluidic biosensor. Lab Chip 8(11), 1847–1851 (2008). https://doi.org/10.1039/B811150B
Browne, A.W., Ramasamy, L., Cripe, T.P., Ahn, C.H.: A lab-on-a-chip for rapid blood separation and quantification of hematocrit and serum analytes. Lab Chip 11(14), 2440–2446 (2011). https://doi.org/10.1039/C1LC20144A
Ballenger, T.F., White, J.L.: An experimental study of flow patterns in polymer fluids in the reservoir of a capillary rheometer. Chem. Eng. Sci. 25(7), 1191–1195 (1970)
Boger, D., Murthy, A.R.: Flow of viscoelastic fluids through an abrupt contraction. Rheol. Acta 11(1), 61–69 (1972)
Tan, K., Tiu, C.: Entry flow behaviour of viscoelastic fluids in an annulus. J. Non-Newton. Fluid Mech. 3(1), 25–40 (1977)
Cable, P., Boger, D.: A comprehensive experimental investigation of tubular entry flow of viscoelastic fluids: Part I. Vortex characteristics in stable flow. AICHE J. 24(5), 869–879 (1978)
Thien, N.P., Tanner, R.I.: A new constitutive equation derived from network theory. J. Non-Newton. Fluid Mech. 2(4), 353–365 (1977)
Phan-Thien, N.: A nonlinear network viscoelastic model. J. Rheol. 22(3), 259–283 (1978)
Gunter, S., Townsend, P., Webster, M.: The simulation of some model viscoelastic extensional flows. Int. J. Numer. Meth. Fluids 23(7), 691–710 (1996)
Xue, S.-C., Phan-Thien, N., Tanner, R.: Numerical study of secondary flows of viscoelastic fluid in straight pipes by an implicit finite volume method. J. Non-Newton. Fluid Mech. 59(2), 191–213 (1995)
Tomé, M., Paulo, G., Pinho, F., Alves, M.: Numerical solution of the PTT constitutive equation for unsteady three-dimensional free surface flows. J. Non-Newton. Fluid Mech. 165(5), 247–262 (2010)
Akyildiz, F.: Dispersion of a solute in a Poiseuille flow of a viscoelastic fluid. Int. j. Eng. Sci. 40(8), 859–872 (2002)
Alves, M.A., Pinho, F.T., Oliveira, P.J.: Study of steady pipe and channel flows of a single-mode Phan-Thien–Tanner fluid. J. Non-Newton. Fluid Mech. 101(1), 55–76 (2001)
Oliveira, P.J., Pinho, F.T.: Analytical solution for fully developed channel and pipe flow of Phan-Thien–Tanner fluids. J. Fluid Mech. 387, 271–280 (1999)
Pinho, F.T., Oliveira, P.J.: Axial annular flow of a nonlinear viscoelastic fluid–an analytical solution. J. Non-Newton. Fluid Mech. 93(2), 325–337 (2000)
Mirzazadeh, M., Escudier, M., Rashidi, F., Hashemabadi, S.: Purely tangential flow of a PTT-viscoelastic fluid within a concentric annulus. J. Non-Newton. Fluid Mech. 129(2), 88–97 (2005)
Das, S., Chakraborty, S.: Analytical solutions for velocity, temperature and concentration distribution in electroosmotic microchannel flows of a non-Newtonian bio-fluid. Anal. Chim. Acta 559(1), 15–24 (2006)
Chakraborty, S.: Electroosmotically driven capillary transport of typical non-Newtonian biofluids in rectangular microchannels. Anal. Chim. Acta 605(2), 175–184 (2007)
Zhao, M., Wang, S., Wei, S.: Transient electro-osmotic flow of Oldroyd-B fluids in a straight pipe of circular cross section. J. Non-Newton. Fluid Mech. 201, 135–139 (2013)
Wang, S., Zhao, M., Li, X.: Transient electro-osmotic flow of generalized Maxwell fluids in a straight pipe of circular cross section. Cent. Eur. J. Phys. 12(6), 445–451 (2014)
Wang, S., Zhao, M.: Analytical solution of the transient electro-osmotic flow of a generalized fractional Maxwell fluid in a straight pipe with a circular cross-section. Eur. J. Mech.-B/Fluids 54, 82–86 (2015)
Escandon, J., Jimenez, E., Hernández, C., Bautista, O., Mendez, F.: Transient electroosmotic flow of Maxwell fluids in a slit microchannel with asymmetric zeta potentials. Eur. J. Mech.-B/Fluids 53, 180–189 (2015)
Afonso, A., Alves, M., Pinho, F.: Analytical solution of mixed electro-osmotic/pressure driven flows of viscoelastic fluids in microchannels. J. Non-Newton. Fluid Mech. 159(1), 50–63 (2009)
Bird, R., Dotson, P., Johnson, N.: Polymer solution rheology based on a finitely extensible bead–spring chain model. J. Non-Newton. Fluid Mech. 7(2), 213–235 (1980)
Dhinakaran, S., Afonso, A., Alves, M., Pinho, F.: Steady viscoelastic fluid flow between parallel plates under electro-osmotic forces: Phan-Thien–Tanner model. J. Colloid Interface Sci. 344(2), 513–520 (2010)
Afonso, A., Alves, M., Pinho, F.: Electro-osmotic flow of viscoelastic fluids in microchannels under asymmetric zeta potentials. J. Eng. Math. 71(1), 15–30 (2011)
Sousa, J., Afonso, A., Pinho, F., Alves, M.: Effect of the skimming layer on electro-osmotic–Poiseuille flows of viscoelastic fluids. Microfluid. Nanofluid. 10(1), 107–122 (2011)
Zhang, Y., Wong, T.N., Yang, C., Ooi, K.T.: Electroosmotic flow in irregular shape microchannels. Int. J. Eng. Sci. 43(19), 1450–1463 (2005)
Bera, S., Bhattacharyya, S.: Electroosmotic flow in the vicinity of a conducting obstacle mounted on the surface of a wide microchannel. Int. J. Eng. Sci. 94, 128–138 (2015)
Ren, L., Li, D.: Electroosmotic flow in heterogeneous microchannels. J. Colloid Interface Sci. 243(1), 255–261 (2001)
Yang, D.Y.: Analytical solution of mixed electroosmotic and pressure-driven flow in rectangular microchannels. Key Eng. Mat. 483, 679–683 (2011)
Ooi, K., Yang, C., Chai, J., Wong, T.: Developing electro-osmotic flow in closed-end micro-channels. Int. J. Eng. Sci. 43(17), 1349–1362 (2005)
Yang, C., Wong, T.N., Ooi, K.T.: Dynamic aspects of electroosmotic flow in rectangular microchannels. Int. J. Eng. Sci. 42(13), 1459–1481 (2004)
Kang, Y., Yang, C., Huang, X.: Analysis of the electroosmotic flow in a microchannel packed with homogeneous microspheres under electrokinetic wall effect. Int. J. Eng. Sci. 42(19), 2011–2027 (2004)
Tang, G., Ye, P., Tao, W.: Pressure-driven and electroosmotic non-Newtonian flows through microporous media via lattice Boltzmann method. J. Non-Newton. Fluid Mech. 165(21), 1536–1542 (2010)
Zimmerman, W., Rees, J., Craven, T.: Rheometry of non-Newtonian electrokinetic flow in a microchannel T-junction. Microfluid. Nanofluid. 2(6), 481–492 (2006)
Bryce, R., Freeman, M.: Abatement of mixing in shear-free elongationally unstable viscoelastic microflows. Lab Chip 10(11), 1436–1441 (2010)
Vakili, M.A., Sadeghi, A., Saidi, M.H.: Pressure effects on electroosmotic flow of power-law fluids in rectangular microchannels. Theor. Comput. Fluid Dyn. 28(4), 409–426 (2014)
Vakili, M.A., Sadeghi, A., Saidi, M.H., Mozafari, A.A.: Electrokinetically driven fluidic transport of power-law fluids in rectangular microchannels. Colloids Surf. A: Physicochem. Eng. Asp. 414, 440–456 (2012)
Huang, Y., Chen, J., Wong, T., Liow, J.-L.: Experimental and theoretical investigations of non-Newtonian electro-osmotic driven flow in rectangular microchannels. Soft Matter 12(29), 6206–6213 (2016)
Afonso, A., Pinho, F., Alves, M.: Electro-osmosis of viscoelastic fluids and prediction of electro-elastic flow instabilities in a cross slot using a finite-volume method. J. Non-Newton. Fluid Mech. 179, 55–68 (2012)
Park, H., Lee, W.: Effect of viscoelasticity on the flow pattern and the volumetric flow rate in electroosmotic flows through a microchannel. Lab Chip 8(7), 1163–1170 (2008)
Yang, C., Li, D.: Electrokinetic effects on pressure-driven liquid flows in rectangular microchannels. J. Colloid Interface Sci. 194(1), 95–107 (1997)
Masliyah, J.H., Bhattacharjee, S.: Electrokinetic and Colloid Transport Phenomena. John Wiley & Sons, Hoboken (2006)
Oliveira, P.: An exact solution for tube and slit flow of a FENE-P fluid. Acta Mech. 158(3–4), 157–167 (2002)
Cruz, D., Pinho, F., Oliveira, P.: Analytical solutions for fully developed laminar flow of some viscoelastic liquids with a Newtonian solvent contribution. J. Non-Newton. Fluid Mech. 132(1), 28–35 (2005)
Park, H., Lee, W.: Helmholtz–Smoluchowski velocity for viscoelastic electroosmotic flows. J. Colloid Interface Sci. 317(2), 631–636 (2008)
Pletcher, R.H., Tannehill, J.C., Anderson, D.: Computational Fluid Mechanics and Heat Transfer. CRC Press, Boca Raton (2012)
Hoffmann, K.A.: Computational Fluid Dynamics for Engineers. Engineering Education System, Austin, TX (1989)
Lam, Y., Chen, X., Yang, C.: Depthwise averaging approach to cross-stream mixing in a pressure-driven microchannel flow. Microfluid. Nanofluid. 1(3), 218–226 (2005)
Sousa, P., Pinho, F., Oliveira, M., Alves, M.: Efficient microfluidic rectifiers for viscoelastic fluid flow. J. Non-Newton. Fluid Mech. 165(11), 652–671 (2010)
Azaiez, J., Guenette, R., Ait-Kadi, A.: Numerical simulation of viscoelastic flows through a planar contraction. J. Non-Newton. Fluid Mech. 62(2), 253–277 (1996)
Vakili, M.A., Sadeghi, A., Saidi, M.H.: Pressure effects on electroosmotic flow of power-law fluids in rectangular microchannels. Theor. Comput. Fluid Dyn. 28, 1–18 (2014)
Probstein, R.F.: Physicochemical Hydrodynamics: An Introduction. John Wiley & Sons, Hoboken (2005)
Author information
Authors and Affiliations
Corresponding author
Additional information
Communicated by Tim Phillips.
Rights and permissions
About this article
Cite this article
Reshadi, M., Saidi, M.H. & Ebrahimi, A. Pure axial flow of viscoelastic fluids in rectangular microchannels under combined effects of electro-osmosis and hydrodynamics. Theor. Comput. Fluid Dyn. 32, 1–21 (2018). https://doi.org/10.1007/s00162-017-0428-y
Received:
Accepted:
Published:
Issue Date:
DOI: https://doi.org/10.1007/s00162-017-0428-y