Abstract
Objectives
To evaluate the effect of pre-scan blood glucose levels (BGL) on standardized uptake value (SUV) in 18F-FDG-PET scan.
Methods
A literature review was performed in the MEDLINE, Embase, and Cochrane library databases. Multivariate regression analysis was performed on individual datum to investigate the correlation of BGL with SUVmax and SUVmean adjusting for sex, age, body mass index (BMI), diabetes mellitus diagnosis, 18F-FDG injected dose, and time interval. The ANOVA test was done to evaluate differences in SUVmax or SUVmean among five different BGL groups (< 110, 110–125, 125–150, 150–200, and > 200 mg/dl).
Results
Individual data for a total of 20,807 SUVmax and SUVmean measurements from 29 studies with 8380 patients was included in the analysis. Increased BGL is significantly correlated with decreased SUVmax and SUVmean in brain (p < 0.001, p < 0.001,) and muscle (p < 0.001, p < 0.001) and increased SUVmax and SUVmean in liver (p = 0.001, p = 0004) and blood pool (p = 0.008, p < 0.001). No significant correlation was found between BGL and SUVmax or SUVmean in tumors. In the ANOVA test, all hyperglycemic groups had significantly lower SUVs compared with the euglycemic group in brain and muscle, and significantly higher SUVs in liver and blood pool. However, in tumors only the hyperglycemic group with BGL of > 200 mg/dl had significantly lower SUVmax.
Conclusion
If BGL is lower than 200 mg/dl no interventions are needed for lowering BGL, unless the liver is the organ of interest. Future studies are needed to evaluate sensitivity and specificity of FDG-PET scan in diagnosis of malignant lesions in hyperglycemia.




Similar content being viewed by others
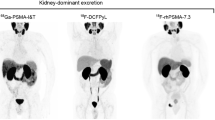
Abbreviations
- FDG-PET:
-
Fluorodeoxyglucose positron emission tomography
- GLUT:
-
Glucose transport protein
- FDG-6-P:
-
F-FDG-6- phosphate
- glucose-6-P:
-
Glucose-6- phosphate
- SNMMI:
-
Society of Nuclear Medicine and Molecular Imaging
- EANM:
-
European Association of Nuclear Medicine
- mg/dl:
-
Milligram per deciliter
- mmol/l:
-
Millimole per liter
- SUV:
-
Standardized uptake values
- SD:
-
Standard deviation
- FBS:
-
Fasting blood sugar
- BMI:
-
Body mass index
- PET/CT:
-
Positron emission tomography / computed tomography
- MD:
-
Mean difference
- CI95%:
-
Confidence interval 95%
- RBC:
-
Red blood cell
References
Moghbel M, Newberg A, Alavi A. Positron emission tomography: ligand imaging. Handb Clin Neurol. 2016;135:229–40.
Basu S, Alavi A. PET-based personalized management in clinical oncology: an unavoidable path for the foreseeable future. PET Clin. 2016;11(3):203–7.
Hustinx R, Benard F, Alavi A. Whole-body FDG-PET imaging in the management of patients with cancer. Semin Nucl Med. 2002;32(1):35–46.
von Schulthess GK, Steinert HC, Hany TF. Integrated PET/CT: current applications and future directions. Radiology. 2006;238(2):405–22.
Rohren EM, Turkington TG, Coleman RE. Clinical applications of PET in oncology. Radiology. 2004;231(2):305–32.
Hess S, et al. The pivotal role of FDG-PET/CT in modern medicine. Acad Radiol. 2014;21(2):232–49.
Sprinz C, et al. Effects of blood glucose level on 18F-FDG uptake for PET/CT in normal organs: a systematic review. PLoS One. 2018;13(2):e0193140.
Volpi S, et al. The role of positron emission tomography in the diagnosis, staging and response assessment of non-small cell lung cancer. Ann Transl Med. 2018;6(5):95.
Heiss WD. Positron emission tomography imaging in gliomas: applications in clinical diagnosis, for assessment of prognosis and of treatment effects, and for detection of recurrences. Eur J Neurol. 2017;24(10):1255–e70.
Rohde M, et al. 18F-fluoro-deoxy-glucose-positron emission tomography/computed tomography in diagnosis of head and neck squamous cell carcinoma: a systematic review and meta-analysis. Eur J Cancer. 2014;50(13):2271–9.
Wu CX, Zhu ZH. Diagnosis and evaluation of gastric cancer by positron emission tomography. World J Gastroenterol. 2014;20(16):4574–85.
Fischer BM, Mortensen J. The future in diagnosis and staging of lung cancer: positron emission tomography. Respiration. 2006;73(3):267–76.
Bastiaannet E, et al. The value of FDG-PET in the detection, grading and response to therapy of soft tissue and bone sarcomas; a systematic review and meta-analysis. Cancer Treat Rev. 2004;30(1):83–101.
Vansteenkiste J, et al. Positron-emission tomography in prognostic and therapeutic assessment of lung cancer: systematic review. Lancet Oncol. 2004;5(9):531–40.
Capirci C, et al. Long-term prognostic value of 18F-FDG PET in patients with locally advanced rectal cancer previously treated with neoadjuvant radiochemotherapy. AJR Am J Roentgenol. 2006;187(2):W202–8.
Challapalli A, Aboagye EO. Positron emission tomography imaging of tumor cell metabolism and application to therapy response monitoring. Front Oncol. 2016;6:44.
Gambhir SS, et al. A tabulated summary of the FDG PET literature. J Nucl Med. 2001;42(5 Suppl):1s–93s.
Weber G. Enzymology of cancer cells (first of two parts). N Engl J Med. 1977;296(9):486–92.
Hiraki Y, Rosen OM, Birnbaum MJ. Growth factors rapidly induce expression of the glucose transporter gene. J Biol Chem. 1988;263(27):13655–62.
Denko NC. Hypoxia, HIF1 and glucose metabolism in the solid tumour. Nat Rev Cancer. 2008;8(9):705–13.
Shaw RJ. Glucose metabolism and cancer. Curr Opin Cell Biol. 2006;18(6):598–608.
Wood IS, Trayhurn P. Glucose transporters (GLUT and SGLT): expanded families of sugar transport proteins. Br J Nutr. 2003;89(1):3–9.
Younes M, et al. Wide expression of the human erythrocyte glucose transporter Glut1 in human cancers. Cancer Res. 1996;56(5):1164–7.
Pauwels EK, et al. The mechanism of accumulation of tumour-localising radiopharmaceuticals. Eur J Nucl Med. 1998;25(3):277–305.
Khan N, et al. 18F-fluorodeoxyglucose uptake in tumor. Mymensingh Med J. 2011;20(2):332–42.
Kumar R, et al. Positron emission tomography imaging in evaluation of cancer patients. Indian J Cancer. 2003;40(3):87–100.
Macheda ML, Rogers S, Best JD. Molecular and cellular regulation of glucose transporter (GLUT) proteins in cancer. J Cell Physiol. 2005;202(3):654–62.
Brown RS, Wahl RL. Overexpression of Glut-1 glucose transporter in human breast cancer. An immunohistochemical study. Cancer. 1993;72(10):2979–85.
Medina RA, Owen GI. Glucose transporters: expression, regulation and cancer. Biol Res. 2002;35(1):9–26.
Ishiki M, Klip A. Minireview: recent developments in the regulation of glucose transporter-4 traffic: new signals, locations, and partners. Endocrinology. 2005;146(12):5071–8.
Gould GW, Holman GD. The glucose transporter family: structure, function and tissue-specific expression. Biochem J. 1993;295(Pt 2):329–41.
Niccoli-Asabella A, et al. 18F-FDGPET/CT: diabetes and hyperglycaemia. Nucl Med Rev Cent East Eur. 2013;16(2):57–61.
Cho NH, et al. IDF diabetes atlas: global estimates of diabetes prevalence for 2017 and projections for 2045. Diabetes Res Clin Pract. 2018;138:271–81.
Clement S, et al. Management of diabetes and hyperglycemia in hospitals. Diabetes Care. 2004;27(2):553–91.
Bonaventura A, Montecucco F. Steroid-induced hyperglycemia: an underdiagnosed problem or clinical inertia? A narrative review. Diabetes Res Clin Pract. 2018;139:203–20.
Beyan C, et al. Severe hyperglycemia as a complication of big ICE chemotherapy in a patient with acute myeloblastic leukemia. Haematologia (Budap). 2002;32(4):505–8.
Walker ED. Hyperglycemia. A complication of chemotherapy in children. Cancer Nurs. 1988;11(1):18–22.
Carrasco-Sanchez FJ, et al. Stress-induced hyperglycemia on complications in non-critically elderly hospitalized patients. Rev Clin Esp. 2018;218(5):223–31.
Delbeke D, et al. Procedure guideline for tumor imaging with 18F-FDG PET/CT 1.0. J Nucl Med. 2006;47(5):885–95.
Boellaard R, et al. FDG PET/CT: EANM procedure guidelines for tumour imaging: version 2.0. Eur J Nucl Med Mol Imaging. 2015;42(2):328–54.
Beyer T, Czernin J, Freudenberg LS. Variations in clinical PET/CT operations: results of an international survey of active PET/CT users. J Nucl Med. 2011;52(2):303–10.
Zhao S, et al. Effects of insulin and glucose loading on FDG uptake in experimental malignant tumours and inflammatory lesions. Eur J Nucl Med. 2001;28(6):730–5.
Cerfolio RJ, et al. The maximum standardized uptake values on positron emission tomography of a non-small cell lung cancer predict stage, recurrence, and survival. J Thorac Cardiovasc Surg. 2005;130(1):151–9.
Weber WA, Schwaiger M, Avril N. Quantitative assessment of tumor metabolism using FDG-PET imaging. Nucl Med Biol. 2000;27(7):683–7.
Westerterp M, et al. Quantification of FDG PET studies using standardised uptake values in multi-centre trials: effects of image reconstruction, resolution and ROI definition parameters. Eur J Nucl Med Mol Imaging. 2007;34(3):392–404.
Higgins JPT, Green S (editors). Cochrane Handbook for Systematic Reviews of Interventions Version 5.1.0 [updated March 2011]. The Cochrane Collaboration, 2011. Available from: www.handbook.cochrane.org.
Liberati A, et al. The PRISMA statement for reporting systematic reviews and meta-analyses of studies that evaluate health care interventions: explanation and elaboration. PLoS Med. 2009;6(7):e1000100.
Wells G, Shea B, O'Connell D, Peterson JE, Welch V, Losos M, Tugwell P. The Newcastle-Ottawa Scale (NOS) for assessing the quality of nonrandomised studies in meta-analyses. Ottawa, Ottawa Hospital Research Institute; 2000
World Health Organization. Definition and diagnosis of diabetes mellitus and intermediate hyperglycemia. Geneva, World Health Organisation; 2006.
Viglianti BL. Plasma glucose effect upon regional brain FDG uptake: implications for semi-quantitative image analysis and dementia classification[abstract]. In: 103rd RSNA Scientific Assembly and Annual Meeting; 2017 November 1, Chicago, SSE16-04. 2017.
Caobelli F, et al. Proposal for an optimized protocol for intravenous administration of insulin in diabetic patients undergoing (18)F-FDG PET/CT. Nucl Med Commun. 2013;34(3):271–5.
Lococo F, et al. 18F-fluorodeoxyglucose positron emission tomographic scan in solid-type p-stage-I pulmonary adenocarcinomas: what can produce false-negative results? Eur J Cardiothorac Surg. 2017;51(4):667–73.
Werner RA, et al. Predictive value of FDG-PET in patients with advanced medullary thyroid carcinoma treated with vandetanib. J Nucl Med. 2017;59(5):756–61.
Garcia JR, et al. Influence of subcutaneous administration of rapid-acting insulin in the quality of (18)F-FDG PET/CT studies. Nucl Med Commun. 2014;35(5):459–65.
Cheung MK, et al. False positive positron emission tomography / computed tomography scans in treated head and neck cancers. Cureus. 2017;9(4):e1146.
Lindholm H, et al. The relation between the blood glucose level and the FDG uptake of tissues at normal PET examinations. EJNMMI Res. 2013;3(1):50.
Schildt J, et al. Seasonal temperature changes do not affect cardiac glucose metabolism. Int J Mol Imaging. 2015;2015:916016.
Iwano S, et al. What causes false-negative PET findings for solid-type lung cancer? Lung Cancer. 2013;79(2):132–6.
Boktor RR, et al. Reference range for intrapatient variability in blood-pool and liver SUV for 18F-FDG PET. J Nucl Med. 2013;54(5):677–82.
Keramida G, et al. Quantification of tumour (18) F-FDG uptake: normalise to blood glucose or scale to liver uptake? Eur Radiol. 2015;25(9):2701–8.
Tatci E, et al. The correlation between pre-treatment fluorodeoxyglucose positron emission tomography/computed tomography parameters and clinical prognostic factors in pediatric Hodgkin lymphoma. Mol Imaging Radionucl Ther. 2017;26(1):9–16.
Sancho-Munoz A, et al. Muscle glucose metabolism in chronic obstructive pulmonary disease patients. Arch Bronconeumol. 2014;50(6):221–7.
Viglianti BL, et al. Effect of hyperglycemia on brain and liver (18)F-FDG standardized uptake value (FDG SUV) measured by quantitative positron emission tomography (PET) imaging. Biomed Pharmacother. 2017;88:1038–45.
Bybel B, et al. Increased F-18 FDG intestinal uptake in diabetic patients on metformin: a matched case-control analysis. Clin Nucl Med. 2011;36(6):452–6.
Barwick TD, et al. 18F-FDG PET-CT uptake is a feature of both normal diameter and aneurysmal aortic wall and is not related to aneurysm size. Eur J Nucl Med Mol Imaging. 2014;41(12):2310–8.
Sprinz C, et al. Effects of blood glucose level on 18F fluorodeoxyglucose (18F-FDG) uptake for PET/CT in normal organs: an analysis on 5623 patients. Sci Rep. 2018;8(1):2126.
Rubello D, et al. Variability of hepatic 18F-FDG uptake at interim PET in patients with Hodgkin lymphoma. Clin Nucl Med. 2015;40(8):e405–10.
Mirpour S, Meteesatien P, Khandani AH. Does hyperglycemia affect the diagnostic value of 18F-FDG PET/CT? Rev Esp Med Nucl Imagen Mol. 2012;31(2):71–7.
Harisankar CN, et al. Utility of high fat and low carbohydrate diet in suppressing myocardial FDG uptake. J Nucl Cardiol. 2011;18(5):926–36.
Huang B, et al. Dynamic PET-CT studies for characterizing nasopharyngeal carcinoma metabolism: comparison of analytical methods. Nucl Med Commun. 2012;33(2):191–7.
Janssen MH, et al. Blood glucose level normalization and accurate timing improves the accuracy of PET-based treatment response predictions in rectal cancer. Radiother Oncol. 2010;95(2):203–8.
Hara T, et al. Significance of chronic marked hyperglycemia on FDG-PET: is it really problematic for clinical oncologic imaging? Ann Nucl Med. 2009;23(7):657–69.
Nakamoto Y, et al. Reproducibility of common semi-quantitative parameters for evaluating lung cancer glucose metabolism with positron emission tomography using 2-deoxy-2-[18F]fluoro-D-glucose. Mol Imaging Biol. 2002;4(2):171–8.
Koyama K, et al. Diagnostic usefulness of FDG PET for pancreatic mass lesions. Ann Nucl Med. 2001;15(3):217–24.
Minn H, et al. Lung cancer: reproducibility of quantitative measurements for evaluating 2-[F-18]-fluoro-2-deoxy-D-glucose uptake at PET. Radiology. 1995;196(1):167–73.
Minn H, et al. [18F]fluorodeoxyglucose uptake in tumors: kinetic vs. steady-state methods with reference to plasma insulin. J Comput Assist Tomogr. 1993;17(1):115–23.
Ishizu K, et al. Effects of hyperglycemia on FDG uptake in human brain and glioma. J Nucl Med. 1994;35(7):1104–9.
Lindholm P, et al. Influence of the blood glucose concentration on FDG uptake in cancer--a PET study. J Nucl Med. 1993;34(1):1–6.
Guerin C, et al. The glucose transporter and blood-brain barrier of human brain tumors. Ann Neurol. 1990;28(6):758–65.
Reske SN, et al. Overexpression of glucose transporter 1 and increased FDG uptake in pancreatic carcinoma. J Nucl Med. 1997;38(9):1344–8.
Kato H, et al. Glut-1 glucose transporter expression in esophageal squamous cell carcinoma is associated with tumor aggressiveness. Anticancer Res. 2002;22(5):2635–9.
Yang J, et al. GLUT-1 overexpression as an unfavorable prognostic biomarker in patients with colorectal cancer. Oncotarget. 2017;8(7):11788–96.
Viglianti BL, et al. Effects of tumor burden on reference tissue standardized uptake for PET imaging: modification of PERCIST criteria. Radiology. 2018;287(3):993–1002.
Yamamoto T, et al. Over-expression of facilitative glucose transporter genes in human cancer. Biochem Biophys Res Commun. 1990;170(1):223–30.
Arora KK, Pedersen PL. Functional significance of mitochondrial bound hexokinase in tumor cell metabolism. Evidence for preferential phosphorylation of glucose by intramitochondrially generated ATP. J Biol Chem. 1988;263(33):17422–8.
Forbes GB, Reina JC. Adult lean body mass declines with age: some longitudinal observations. Metabolism. 1970;19(9):653–63.
Gheller BJ, et al. Understanding age-related changes in skeletal muscle metabolism: differences between females and males. Annu Rev Nutr. 2016;36:129–56.
Haizlip KM, Harrison BC, Leinwand LA. Sex-based differences in skeletal muscle kinetics and fiber-type composition. Physiology (Bethesda). 2015;30(1):30–9.
Bogan JS. Regulation of glucose transporter translocation in health and diabetes. Annu Rev Biochem. 2012;81:507–32.
Cline GW, et al. Impaired glucose transport as a cause of decreased insulin-stimulated muscle glycogen synthesis in type 2 diabetes. N Engl J Med. 1999;341(4):240–6.
Kershaw EE, Flier JS. Adipose tissue as an endocrine organ. J Clin Endocrinol Metab. 2004;89(6):2548–56.
Ferrannini E, et al. Effect of fatty acids on glucose production and utilization in man. J Clin Invest. 1983;72(5):1737–47.
Ismail-Beigi F. Metabolic regulation of glucose transport. J Membr Biol. 1993;135(1):1–10.
Marom EM, et al. Correlation of FDG-PET imaging with Glut-1 and Glut-3 expression in early-stage non-small cell lung cancer. Lung Cancer. 2001;33(2–3):99–107.
Yip WCY, et al. Prevalence of pre-diabetes across ethnicities: a review of impaired fasting glucose (IFG) and impaired glucose tolerance (IGT) for classification of dysglycaemia. Nutrients. 2017;9(11).
Simonson GD, Kendall DM. Diagnosis of insulin resistance and associated syndromes: the spectrum from the metabolic syndrome to type 2 diabetes mellitus. Coron Artery Dis. 2005;16(8):465–72.
Slieker LJ, et al. Glucose transporter levels in tissues of spontaneously diabetic Zucker fa/fa rat (ZDF/drt) and viable yellow mouse (Avy/a). Diabetes. 1992;41(2):187–93.
Kelley DE, et al. The effect of non-insulin-dependent diabetes mellitus and obesity on glucose transport and phosphorylation in skeletal muscle. J Clin Invest. 1996;97(12):2705–13.
Kelley DE, Williams KV, Price JC. Insulin regulation of glucose transport and phosphorylation in skeletal muscle assessed by PET. Am J Phys. 1999;277(2 Pt 1):E361–9.
Pardridge WM, Boado RJ, Farrell CR. Brain-type glucose transporter (GLUT-1) is selectively localized to the blood–brain barrier. Studies with quantitative western blotting and in situ hybridization. J Biol Chem. 1990;265(29):18035–40.
Vannucci SJ, Maher F, Simpson IA. Glucose transporter proteins in brain: delivery of glucose to neurons and glia. Glia. 1997;21(1):2–21.
Adeva-Andany MM, et al. Liver glucose metabolism in humans. Biosci Rep. 2016;36(6):e00416.
Ferrannini E, et al. The disposal of an oral glucose load in healthy subjects. A quantitative study. Diabetes. 1985;34(6):580–8.
Woerle HJ, et al. Pathways for glucose disposal after meal ingestion in humans. Am J Physiol Endocrinol Metab. 2003;284(4):E716–25.
Adeva-Andany MM, et al. Glycogen metabolism in humans. BBA Clin. 2016;5:85–100.
McDevitt RM, et al. De novo lipogenesis during controlled overfeeding with sucrose or glucose in lean and obese women. Am J Clin Nutr. 2001;74(6):737–46.
Karim S, Adams DH, Lalor PF. Hepatic expression and cellular distribution of the glucose transporter family. World J Gastroenterol. 2012;18(46):6771–81.
Michels NA. Newer anatomy of the liver and its variant blood supply and collateral circulation. Am J Surg. 1966;112(3):337–47.
Selle D, et al. Analysis of vasculature for liver surgical planning. IEEE Trans Med Imaging. 2002;21(11):1344–57.
Joost HG, Thorens B. The extended GLUT-family of sugar/polyol transport facilitators: nomenclature, sequence characteristics, and potential function of its novel members (review). Mol Membr Biol. 2001;18(4):247–56.
Harik SI, Behmand RA, Arafah BM. Chronic hyperglycemia increases the density of glucose transporters in human erythrocyte membranes. J Clin Endocrinol Metab. 1991;72(4):814–8.
Bertoldo A, et al. Interactions between delivery, transport, and phosphorylation of glucose in governing uptake into human skeletal muscle. Diabetes. 2006;55(11):3028–37.
James DE. Targeting of the insulin-regulatable glucose transporter (GLUT-4). Biochem Soc Trans. 1994;22(3):668–70.
Roy FN, et al. Impact of intravenous insulin on 18F-FDG PET in diabetic cancer patients. J Nucl Med. 2009;50(2):178–83.
Acknowledgments
This research study was not supported by any specific grant from funding agencies in the public, commercial, or not-for-profit sectors.
We would like to acknowledge Abdullah Al-Zaghal and Thomas J. Werner for their contributions to the revised version of this manuscript.
Author information
Authors and Affiliations
Corresponding author
Ethics declarations
Conflict of interest
All the authors confirm that there is no conflict of interest to declare. This paper has received no grant from any funding source.
Ethical approval
All procedures performed in studies involving human participants were in accordance with the ethical standards of the institutional and/or national research committee and with the 1964 Helsinki Declaration and its later amendments or comparable ethical standards.
Informed consent
Informed consent was obtained from all individual participants included in the study.
Rights and permissions
About this article
Cite this article
Eskian, M., Alavi, A., Khorasanizadeh, M. et al. Effect of blood glucose level on standardized uptake value (SUV) in 18F- FDG PET-scan: a systematic review and meta-analysis of 20,807 individual SUV measurements. Eur J Nucl Med Mol Imaging 46, 224–237 (2019). https://doi.org/10.1007/s00259-018-4194-x
Received:
Accepted:
Published:
Issue Date:
DOI: https://doi.org/10.1007/s00259-018-4194-x