Abstract
Background
Camostat mesylate, an oral serine protease inhibitor, is a powerful TMPRSS2 inhibitor and has been reported as a possible antiviral treatment against COVID-19. Therefore, we aim to assess the safety and efficacy of camostat mesylate for COVID-19 treatment.
Methods
A systematic review and meta-analysis synthesizing randomized controlled trials from PubMed, Scopus, Embase, Cochrane, Web of Science, clinical trials.gov, and medrxiv until June 2023. The outcomes were pooled using Mean difference (MD) for continuous outcomes and risk ratio (RR) for dichotomous outcomes. The protocol is registered in PROSPERO with ID CRD42023439633.
Results
Nine RCTs, including 1,623 patients, were included in this analysis. There was no difference between camostat mesylate and placebo in producing negative PCR test results at 1–7 days (RR: 0.76, 95% CI: [0.54, 1.06] P = 0.1), 8–14 days (RR: 1.02, 95% CI: [0.84, 1.23] P = 0.87), or 15–21 days (RR: 0.99, 95% CI: [0.82, 1.19] P = 0.90); clinical resolution of symptoms at 1–7 days (RR: 0.94 (95% CI: 0.58, 1.53) P = 0.81), 8–14 days (RR: 0.91, 95% CI: [0.74, 1.11] P = 0.33, ), or 15–21 days (RR: 0.77, 95% CI: [0.40, 1.51] P = 0.45); and time to symptom improvement (MD:-0.38 weeks (95% CI: [-1.42, 0.66] P = 0.47, I2 = 85%).
Conclusion
Camostat mesylate did not improve clinical outcomes in patients with COVID-19, compared to placebo.
Similar content being viewed by others
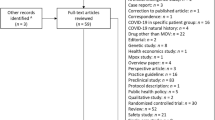
Introduction
Coronavirus disease 2019 (COVID-19) is a novel coronavirus that originated in China’s Hubei region and spread throughout the world in late 2019 [1,2,3]. On March 11th, 2020, the WHO classified COVID-19 as a pandemic. COVID-19 is extremely contagious and has put an enormous burden on healthcare systems around the world. Pharmacological treatment of infected patients is required until herd immunity is acquired by extensive viral outbreaks or an effective prophylactic vaccination, since social distance is not an effective long-term stand-alone method.
Current treatment of COVID-19 is primarily hospital-based and directed at advanced disease, for example with remdesivir with FDA approval based on three pivotal trials [4,5,6,7], and corticosteroids such as dexamethasone [8, 9]. Furthermore, Monoclonal antibodies can be used in the outpatient setting but they are expensive, logistically challenging to administer, and have variable degrees of efficacy due to viral variants [9].
Despite the recent progress of antiviral drugs, further therapeutic alternatives are still required, especially for post-exposure prophylaxis and COVID-19 early treatment in outpatient settings. New pharmaceutical targets have been suggested as viable options for antiviral drugs against COVID-19. To clarify, viral replication and disease progression can be effectively stopped by blocking viral host cell entry. Previous experimental data [10,11,12] show that the SARS-CoV-2 spike (S) protein binds to target cells via the host cell factors angiotensin-converting enzyme 2 (ACE-2) and that S protein cleavage by the host cell surface trans-membrane protease serine 2 (TMPRSS2) allow entry into target cells.
Camostat mesylate has been used in clinical settings to treat pancreatitis and reflux esophagitis for over two decades [11,12,13]. Camostat mesylate molecules inhibit TMPRSS2 priming of S protein, a process that has been demonstrated to be both essential and sufficient for viral entry into respiratory epithelial cells [11, 12]. Also, COVID-19 infection of primary human lung epithelial cells was demonstrated to be inhibited by camostat mesylate. Camostat mesylate is a prodrug that, upon entering the bloodstream, rapidly converts to the pharmacologically active metabolite FOY-251, which inhibits TMPRSS2. FOY-251 has an EC50 of 178 nM against SARS-CoV-2 infection in Calu-3 lung cell culture [11]. Moreover, even at high dosages, it has few, mild adverse effects and is readily produced at low costs. Hence, camostat mesylate was predicted to be a good candidate for the treatment of COVID-19. This systematic review and meta-analysis aims to synthesize evidence from randomized controlled trials (RCTs), investigating the efficacy and safety of camostat mesylate for COVID-19 treatment.
Methodology
Protocol Registration
The Preferred Reporting Items for Meta-Analyses according to (PRISMA) guidelines [14] were followed for this meta-analysis. Our protocol was prospectively registered in the International Prospective Register of Systematic Reviews (PROSPERO) with ID CRD42023439633.
Data source and search strategy
An electronic search of PubMed, Scopus, Embase, Cochrane, Web of Science, clinical trials.gov, and medrxiv was conducted from inception to June 2023 without any search restrictions. In addition, references from any retrieved trials were screened manually to identify potentially relevant articles. Further details regarding data source and search strategy are given in (Table S1).
Eligibility criteria
A PICO criterion was used to include RCTs: population (P): patients with COVID-19 regardless of the disease severity; intervention (I): camostat mesylate; control (C): placebo with or without the standard of care; and outcomes (O): primary outcomes of this review were the efficacy outcomes: all-cause mortality, PCR negative, clinical resolution of symptoms, time to symptom improvement, hospitalization duration, and intensive care unit (ICU) admission or mechanical ventilation. The secondary outcomes included safety outcomes: any adverse events, any serious adverse, elevated liver enzymes, and specific safety events.
Study selection
Three reviewers (A.I., S.R., & M.M.) independently screened the studies using Covidence [15] after duplicates were screened and removed automatically. The remaining studies were carefully assessed in accordance with the eligibility criteria. All studies were initially short-listed based on title and abstract, and subsequently, full-length articles were reviewed. Any discrepancies and conflicts between the selected studies were resolved by a U.K.
Data extraction
Four reviewers (A.I., S.R., M.M., & M.M.N.) extracted data independently, including baseline, efficacy, and safety data. Baseline data included number of participants in each, mean age, gender, mean body mass index (BMI), mean duration of symptoms, ordinal severity score, and comorbidity data. Efficacy data was recorded in terms of number of patients with negative PCR (at 1–7 days, 8–14 days, and 15–21 days or more), clinical resolution of symptoms (at 1–7 days, 8–14 days, and 15–21 days or more), time to improvement in symptoms, viral load at the end of follow up, duration of hospitalization, all-cause mortality, and ICU admission or mechanical ventilation. Safety data included the incidence of any adverse event, any serious adverse event, and specific adverse events. Conflicts were solved by mutual discussion between reviewers.
Risk of Bias and Certainty of evidence
Four reviewers (A.I., S.R., M.M., & F.S) independently assessed the quality of included studies using the modified Cochrane Collaboration’s risk of bias tool for randomized controlled trials [16] Conflicts were solved by mutual discussion between reviewers.
To appraise the quality of evidence, two reviewers (M.A. and U.K.) utilized the Grading of Recommendations Assessment, Development, and Evaluation (GRADE) guidelines [17, 18]. We considered inconsistency, imprecision, indirectness, publication bias, and risk of bias. The evaluation was carried out for each outcome, and the decisions made were justified and documented. Any discrepancies were settled through discussion.
We followed the confidence interval cutoffs provided by Cochrane consumers and communication “how to grade?” guidelines [19].
Statistical analysis
RevMan (version 5.3; Copenhagen: The Nordic Cochrane Centre, The Cochrane Collaboration, 2014) was used for all statistical analyses [20]. The results from trials were presented as risk ratios (RR) for dichotomous outcomes and mean difference (MD) for continuous outcomes with a 95% confidence interval (CI) and were pooled using a fixed-effects model in case of homogenous data and random effects model in case of heterogeneous data. According to the Cochrane Handbook (chapter nine) [21]., heterogeneity was considered significant if the alpha value of the Chi-square test is below 0.1, while the interpretation of the I-square test is as follows: (0–40%) not significant, (30–60%) moderate heterogeneity, (50–90%) substantial heterogeneity, and (75–100%) considerable heterogeneity.
Results
Search results and study characteristics
The initial literature search yielded 816 studies after the removal of duplicate (n = 151) and irrelevant studies (n = 656), leaving nine RCTs for inclusion in the final quantitative and qualitative analysis. Out of total, 63 studies were excluded in full text screening with reason of exclusion mentioned in (Table S2). Finally, nine studies were included in the final analysis. Further details can be obtained from the PRISMA flowchart in (Fig. 1).
Included studies characteristics
Nine RCTs [22,23,24,25,26,27,28,29,30] were included in the final analysis with a total of 1,623 participants (n = 912 in the camostat mesylate group and n = 711 in the placebo group), with 52.7% of the patients being male. Most of the studies were conducted in the USA (n = 4), followed by an equal number of trials from Sweden, Austria, Japan, Denmark, Belgium, and South Korea. Camostat mesylate and placebo were given as oral tablets. The mean duration of follow-up was 2.8 weeks. The definition and criteria for serious adverse events were different in each article so we have explained it in Table S3 to make it clear. Further information about baseline study and patient characteristics are available in (Tables 1, 2), respectively.
Risk of Bias and Certainty of evidence
After a careful assessment using the Cochrane ROB 2.0 tool, six RCTs were concluded as having a low risk of bias [22,23,24,25,26,27], two showing some concerns [28, 30], and one with a high risk of bias [29].(Fig. 2). Certainty of evidence is demonstrated in detail in a GRADE evidence profile (Table 3). The details of all the domains which are assessed are mentioned in (Table S4-S12).
Quality assessment of risk of bias in the included trials. The upper panel presents a schematic representation of risks (low = red, unclear = yellow, and high = red) for specific types of biases of each of the studies in the review. The lower panel presents risks (low = red, unclear = yellow, and high = red) for the subtypes of biases of the combination of studies included in this review
Efficacy outcomes
All-cause mortality
The analysis showed an insignificant difference between camostat mesylate and the placebo groups, and no significant heterogeneity was observed (RR: 0.55, 95% CI: [0.27, 1.10] P = 0.09, I2 = 31%) (Fig. 3-A).
Negative PCR
There was no difference between both groups at 1–7 days (RR: 0.77, 95% CI: [0.55, 1.07] P = 0.12, I2 = 0%), 8–14 days (RR: 1.03, 95% CI: [0.85, 1.24] P = 0.80, I2 = 0%), and 15–21 days (RR: 1.04, 95% CI: [0.91, 1.20] P = 0.52, I2 = 33%), without any observed significant heterogeneity (Fig. 3-B).
Clinical resolution of symptoms
There was no difference between both groups at 1–7 days (RR: 1.02, 95% CI: [0.78, 1.34] P = 0.87, I2 = 49%), 8–14 days (RR: 0.90, 95% CI: [0.73, 1.10] P = 0.30, I2 = 0%), and 15–21 days (RR: 0.77, 95% CI: [0.40, 1.50] P = 0.45, I2 = 0%) without any observed significant heterogeneity (Fig. 4-A).
Time to Symptom Improvement
There was no difference between both groups (MD: -0.38 weeks, 95% CI: [-1.42, 0.66] P = 0.47, I2 = 85%) (Fig. 4-B). Significantly high heterogeneity was observed (I2 = 85%, P = 0.0002) which was resolved by removing Karolyi et al. by leave-one-out sensitivity analysis (I2 = 0%, P = 0.88) (Table S13).
ICU admission or mechanical ventilation
There was no difference between both groups (RR: 0.55, 95% CI: [0.20, 1.53] P = 0.25, I2 = 57%) (Fig. 4-C). Significant heterogeneity was observed which could not be resolved by a sensitivity analysis (Table S13).
Safety outcomes
There was no difference between both groups regarding the incidence of any adverse events (RR: 0.93, 95% CI: [0.67, 1.29] P = 0.66, I2 = 80%), elevated liver enzymes (RR: 0.30, 95% CI: [0.07, 1.30] P = 0.12, I2 = 0%), abdominal pain (RR: 0.57, 95% CI: [0.19, 1.73] P = 0.32, I2 = 0%), and pruritis (RR: 1.76, 95% CI: [0.43, 7.11] P = 0.43, I2 = 0%). However, compared to the placebo group, the camostat mesylate group showed a significantly higher risk of any serious adverse events (RR: 1.77, 95% CI: [1.1, 2.83] P = 0.02, I2 = 35%), and a lower risk of diarrhea (RR: 0.35, 95% CI: [0.18, 0.67] P = 0.002, I2 = 41%) (Fig. 5). More details about serious adverse events in each study are given in table S13.
Statistically significant heterogeneity was observed in any adverse events outcome (I2 = 80%, p < 0.0001). A leave-one-out sensitivity analysis was conducted; however, no single study could be found responsible for it (Table S13).
Discussion
The present systematic review and meta-analysis showed that camostat mesylate is overall ineffective in improving the clinical outcomes of COVID-19 patients while increasing the risk of any serious adverse events. Hence, camostat mesylate exhibited no superiority to placebo in reducing the risk of mortality and ICU admission or mechanical ventilation events. Similarly, it did not accelerate either the clinical recovery (clinical resolution of symptoms and time to symptom improvement) or the viral clearance (time for PCR negativation). Therefore, the current RCTs-based evidence suggests that camostat mesylate when given as monoantiviral therapy for COVID-19 patients may have no particular utility whether in mild, moderate, or severe forms.
Death in patients with acute SARS-CoV-2 infection results from several causes, including multiple organ dysfunction syndrome, nosocomial superinfection (mainly ventilator-associated pneumonia), refractory hypoxemia/pulmonary fibrosis (secondary to extensive lung damage), and fatal ischemic events affecting venous (e.g., pulmonary embolism) or arterial (e.g., stroke and myocardial infarction) circulation [31]. For an antiviral drug to reduce the risk of these events it should prevent the progression to severe COVID-19 and hospitalization by early eradication of infection such as the FDA-approved drugs’ combination nirmatrelvir/ritonavir (Paxlovid) which is also based on anti-protease activity [32, 33].
Since the use of camostat mesylate did not affect the features of disease progression (infection’s clinical evolution, viral load kinetics, ICU admission, and mechanical ventilation) reduction in mortality rates is unlikely to be achieved. Additionally, the absence of a significant decrease in hospitalization rates signifies that camostat mesylate has low benefits in patients at risk for severe COVID-19. Furthermore, the no change in time for clinical recovery among camostat-treated groups indicates that this drug may be a non-useful strategy to treat COVID-19 outpatients with both moderate and mild forms.
Moreover, the earlier control of viral replication is essential for an antiviral drug to be effective in COVID-19 patients [34]. On one hand, this would prevent the tissular injury induced by either SARS-CoV-2 or its associated inflammation, and on the other hand, it would decrease the infectivity of patients, thereby minimizing disease transmission. The anti-SARS-CoV-2 activity of camostat mesylate was speculated from its potential to block TMPRSS2-mediated viral fusion; thus, inhibiting viral replication in host cells, as shown by in vitro human cell and animal studies [35, 36]. The fact that camostat mesylate did not induce significant acceleration in PCR negativation time likely reflects its failure to effectively contribute to viral clearance and replication arrest/prevention.
Mechanistically, this seems to be due to two main reasons: (i) the non-pharmacological effectiveness of camostat mesylate as a TMPRSS2 inhibitor administered in monotherapy, or (ii) the non-utility of TMPRSS2 inhibition as an exclusive strategy to prevent viral invasion (the most likely probability). Hence, studies on the molecular pharmacology of camostat mesylate indicated that it may not be the optimal ligand to block TMPRSS2 activity [37,38,39]. Notably, it has been revealed that camostat has lesser inhibition potential compared to a similar TMPRSS2 blocker nafamostat as the latter forms significantly higher amounts of enzyme-substrate stable complexes [39]. Remarkably, the pharmacological potency of camostat mesylate was shown to be 10-fold less than that of nafamostat mesylate [2]. Further results from animal studies concluded that nafamostat is a better candidate for the prevention of SARS-CoV-2 TMPRSS2-mediated entry compared to camostat [40]. Simultaneously, it has been recently demonstrated that SARS-CoV-2 can enter target cells without the need for ACE2 and TMPRSS2 participation through “cell-to-cell fusion” mechanism. Notably, the involvement of TMPRSS2 in this mechanism was found to be dispensable suggesting that SARS-CoV-2 exhibits TMPRSS2-independent cellular invasion strategies [41].
Moreover, even in the absence of TMPRSS2, SARS-CoV-2 has an alternative route of entry by endocytosis and transportation into endolysosomes where it is released to the cytosol via the action of acid-activated cathepsin L protease [42]. Therefore, SARS-CoV-2 can use these pathways to escape from camostat mesylate and other specific inhibitors of TMPRSS2. This possibility is more pronounced with the novel SARS-CoV-2 variants (i.e., Omicron) which no longer rely on TMPRSS-2 as a fusogenicity factor [43]. Consequently, targeting TMPRSS2 alone is not sufficient to fully prevent penetration of SARS-CoV-2 to host cells. Another potential disadvantage of targeting TMPRSS2 is that this protein displays an interindividual structural variability with some functional variants being expressed at relatively high frequencies among many human populations [44]. There are also interindividual quantitative variations in TMPRSS2 levels secondary to genetic polymorphisms across populations [45]. Both qualitative and quantitative variations in TMPRSS2 may alter the individuals’ response to camostat mesylate and similar drugs by potentially decreasing ligand potency and efficiency.
Besides the low efficacy profile, analysis of the safety profile indicated some concerns with camostat mesylate due to a higher risk of any serious adverse events in the treated groups compared to controls. The mechanisms of this molecule’s toxicity are unclear; however, since TMPRSS2 is ubiquitously expressed in the human body its inhibition may result in systemic undesirable effects. Additionally, camostat mesylate has a broad action on other proteases involved in multiple functions such as blood pressure control and renal function, inflammation, and coagulation [46]; which when inhibited in COVID-19 patients (especially those with severe forms) may lead to more harms than goods. Worth mentioning that camostat mesylate has anti-diarrheic effects as it was shown to normalize intestinal hyperpermeability in rats which could explain the lower susceptibility to diarrhea in COVID-19 patients compared to placebo [43].
Strengths and limitations
To the best of our knowledge, this is the first meta-analysis that assesses the safety and efficacy of camostat mesylate in COVID-19 patients. Therefore, this paper presents the gold-standard evidence on this topic including all available RCTs that met our criteria to reach the highest accessible quality of evidence. We analyzed data from a large number (n = 1,623) of patients and provided key findings. However our paper is undermined by the following: first, we included three non-peer-reviewed reports, including a preprint ref and two unpublished RCTs data [27, 30]. Second, the included studies suffered from significant heterogeneity in the camostat dosing regimen, which can affect our findings. Third, all the included studies recruited patients with mild to moderate COVID-19, with only Gunst et al. and Karolyi et al. [26, 29], recruiting hospitalized patients with moderate to severe disease; therefore, our results may not be generalizable for severe COVID-19.
Implications and future perspectives
Targeting viral entry is a well-established strategy to fight viral diseases such as HIV and influenza virus infections; however, its benefit in COVID-19 remains questionable and is not yet supported by robust quality of evidence. Until full data becomes available, the results in this study do not exclude the usefulness of camostat mesylate in the context of COVID-19 infection as co-administration with other synergistic antiviral drugs may boost its efficacy profile. Since furin, another transmembranous enzyme involved in the proteolytic processing of SARS-CoV-2 is necessary for TMPRSS2-independent fusion (i.e., cell-to-cell fusion), the combination of furin and TMPRSS2 inhibitors may enhance the overall preventive effects on viral entry and infectivity [13, 38]. Nevertheless, the constant changes in SARS-CoV-2 cellular invasion pathways may not facilitate the development of the most adequate combination for viral entry inhibitors. Importantly, the presence of safety concerns with camostat mesylate use among COVID-19 patients should justify more caution and strict patient monitoring in future evaluations. Based on these concerns and the lack of proof of effectiveness, current guidelines should recommend against the use of camostat mesylate in COVID-19 patients outside the context of clinical trials.
Conclusion
The current evidence does not support the efficacy of camostat mesylate in treating COVID-19 infection. Rather, it indicates some safety concerns that should be considered before further testing this drug in large-scale trials. Nevertheless, since the available data is incomplete more RCTs are still required to conclude the therapeutic benefit of camostat mesylate in COVID-19. At the same time, it might also be worthy to continue investigating the utility of viral entry inhibitors as potential treatment for COVID-19 by focusing on other TMPRSS2 inhibitors with greater pharmacological potency, agents with TMPRSS2-independent activity, or effective synergistic combinations of both.
Data availability
All data generated or analysed during this study are included in this published article [and its supplementary information files].
References
Khan S et al. Emergence of a novel coronavirus, severe Acute Respiratory Syndrome Coronavirus 2: Biology and Therapeutic options. J Clin Microbiol, 2020. 58(5).
Deslandes A, et al. SARS-CoV-2 was already spreading in France in late December 2019. Int J Antimicrob Agents. 2020;55(6):106006.
Lake MA. What we know so far: COVID-19 current clinical knowledge and research. Clin Med (Lond). 2020;20(2):124–7.
Beigel JH, et al. Remdesivir for the treatment of Covid-19 - final report. N Engl J Med. 2020;383(19):1813–26.
Goldman JD, et al. Remdesivir for 5 or 10 days in patients with severe Covid-19. N Engl J Med. 2020;383(19):1827–37.
Horby P, et al. Dexamethasone in hospitalized patients with Covid-19. N Engl J Med. 2021;384(8):693–704.
Spinner CD, et al. Effect of Remdesivir vs Standard Care on Clinical Status at 11 days in patients with moderate COVID-19: a Randomized Clinical Trial. JAMA. 2020;324(11):1048–57.
Johnson RM, Vinetz JM. Dexamethasone in the management of covid – 19. BMJ. 2020;370:m2648.
Verderese JP, et al. Neutralizing monoclonal antibody treatment reduces hospitalization for mild and moderate coronavirus Disease 2019 (COVID-19): a real-world experience. Clin Infect Dis. 2022;74(6):1063–9.
Kreutzberger AJB, et al. Synergistic block of SARS-CoV-2 infection by Combined Drug Inhibition of the host entry factors PIKfyve kinase and TMPRSS2 protease. J Virol. 2021;95(21):e0097521.
Hoffmann M, et al. Camostat mesylate inhibits SARS-CoV-2 activation by TMPRSS2-related proteases and its metabolite GBPA exerts antiviral activity. EBioMedicine. 2021;65:103255.
Hoffmann M et al. Nafamostat Mesylate blocks activation of SARS-CoV-2: New Treatment option for COVID-19. Antimicrob Agents Chemother, 2020. 64(6).
Motoo Y. Antiproteases in the treatment of chronic pancreatitis. Jop. 2007;8(4 Suppl):533–7.
Page MJ, et al. The PRISMA 2020 statement: an updated guideline for reporting systematic reviews. BMJ. 2021;372:n71.
McKeown S, Mir ZM. Considerations for conducting systematic reviews: evaluating the performance of different methods for de-duplicating references. Syst Rev. 2021;10(1):38.
Higgins JP, et al. The Cochrane collaboration’s tool for assessing risk of bias in randomised trials. BMJ. 2011;343:d5928.
Guyatt GH, et al. What is quality of evidence and why is it important to clinicians? BMJ. 2008;336(7651):995–8.
Guyatt GH, et al. GRADE: an emerging consensus on rating quality of evidence and strength of recommendations. BMJ. 2008;336(7650):924–6.
Cochrane Consumers and Communication, https://cccrg.cochrane.org/author-resources
Reyman J. Book review: St.amant, Kirk, & Zemliansky, Pavel, editors. (2005). internet-based workplace communications: Industry and academic applications. hershey, PA: Information science publishing. Journal of Business and Technical Communication. 2007;21(4):442–5. doi:10.1171.
Higgins JP, Green S. Cochrane handbook for systematic reviews of interventions 2008.
Chupp G, et al. A phase 2 Randomized, Double-Blind, placebo-controlled trial of oral Camostat Mesylate for Early Treatment of COVID-19 outpatients showed shorter illness course and attenuation of loss of smell and taste. medRxiv; 2022.
Jilg N, et al. One week of oral Camostat Versus Placebo in Nonhospitalized adults with mild-to-moderate coronavirus Disease 2019: a randomized controlled phase 2 trial. Clin Infect Dis. 2023;77(7):941–9.
Tobback E, et al. Efficacy and safety of camostat mesylate in early COVID-19 disease in an ambulatory setting: a randomized placebo-controlled phase II trial. Int J Infect Dis. 2022;122:628–35.
Kinoshita T, et al. A multicenter, double-blind, randomized, parallel-group, placebo-controlled study to evaluate the efficacy and safety of camostat mesilate in patients with COVID-19 (CANDLE study). BMC Med. 2022;20(1):342.
Gunst JD, et al. Efficacy of the TMPRSS2 inhibitor camostat mesilate in patients hospitalized with Covid-19-a double-blind randomized controlled trial. EClinicalMedicine. 2021;35:100849.
Camostat Efficacy vs. Placebo for Outpatient Treatment of COVID-19 (CAMELOT) clinicaltrials.govhttps://classic.clinicaltrials.gov/ct2/history/NCT04583592?V_9=View.
Kim YS, et al. A Double-Blind, randomized, Placebo-Controlled, phase II clinical study to evaluate the efficacy and safety of Camostat Mesylate (DWJ1248) in adult patients with mild to moderate COVID-19. Antimicrob Agents Chemother. 2023;67(1):e0045222.
Karolyi M, et al. Camostat Mesylate Versus Lopinavir/Ritonavir in hospitalized patients with COVID-19-Results from a Randomized, controlled, open label, platform trial (ACOVACT). Front Pharmacol. 2022;13:870493.
Oral Camostat Compared With Standard Supportive Care in Mild-Moderate COVID-19 Patients (COPS-2003) clinicaltrials govhttps://classic.clinicaltrials.gov/ct2/show/NCT04524663.
Amani B, Amani B. Efficacy and safety of nirmatrelvir/ritonavir (paxlovid) for COVID-19: a rapid review and meta-analysis. J Med Virol. 2023;95(2):e28441.
Tian H, et al. Efficacy and safety of paxlovid (nirmatrelvir/ritonavir) in the treatment of COVID-19: an updated meta-analysis and trial sequential analysis. Rev Med Virol. 2023;33(5):e2473.
Hoffmann M et al. Camostat mesylate inhibits SARS-CoV-2 activation by TMPRSS2-related proteases and its metabolite GBPA exerts antiviral activity. bioRxiv, 2020.
Abuelazm M, et al. The Effect of Nitazoxanide on the clinical outcomes in patients with COVID-19: a systematic review and Meta-analysis of Randomized controlled trials. Clin Drug Investig. 2022;42(12):1031–47.
Breining P, et al. Camostat mesylate against SARS-CoV-2 and COVID-19-Rationale, dosing and safety. Basic Clin Pharmacol Toxicol. 2021;128(2):204–12.
Li K, et al. The TMPRSS2 inhibitor Nafamostat reduces SARS-CoV-2 Pulmonary infection in mouse models of COVID-19. mBio. 2021;12(4):e0097021.
Reuter N et al. SARS-CoV-2 spike protein is capable of inducing cell-cell fusions independent from its receptor ACE2 and this activity can be impaired by furin inhibitors or a subset of monoclonal antibodies. Viruses, 2023. 15(7).
Koch J, et al. TMPRSS2 expression dictates the entry route used by SARS-CoV-2 to infect host cells. Embo j. 2021;40(16):e107821.
Meng B, et al. Altered TMPRSS2 usage by SARS-CoV-2 omicron impacts infectivity and fusogenicity. Nature. 2022;603(7902):706–14.
Ortiz-Fernández L, Sawalha AH. Genetic variability in the expression of the SARS-CoV-2 host cell entry factors across populations. Genes Immun. 2020;21(4):269–72.
Li F, et al. Distinct mechanisms for TMPRSS2 expression explain organ-specific inhibition of SARS-CoV-2 infection by enzalutamide. Nat Commun. 2021;12(1):866.
Maekawa A, et al. Camostat mesilate inhibits prostasin activity and reduces blood pressure and renal injury in salt-sensitive hypertension. J Hypertens. 2009;27(1):181–9.
Yuan L, et al. Camostat mesilate inhibits pro-inflammatory cytokine secretion and improves cell viability by regulating MFGE8 and HMGN1 in lipopolysaccharide-stimulated DF-1 chicken embryo fibroblasts. PeerJ. 2021;9:e12053.
Kastenhuber ER et al. Coagulation factors directly cleave SARS-CoV-2 spike and enhance viral entry. Elife, 2022. 11.
Zhao J, et al. A protease inhibitor against acute stress-induced visceral hypersensitivity and paracellular permeability in rats. Eur J Pharmacol. 2011;654(3):289–94.
Assylbekova A, et al. Camostat does not inhibit the proteolytic activity of Neutrophil Serine proteases. Volume 15. Pharmaceuticals (Basel); 2022. 5.
Acknowledgements
None.
Funding
We received no funding for this study.
Author information
Authors and Affiliations
Contributions
M.A. conceived the idea. M.M. and S.R. designed the research workflow. U.K. and A.M. searched the databases. S.R., M.M., and A.M. screened the retrieved records. Four reviewers S.R., M.M., F.S., and A.M. extracted data independently extracted relevant data, assessed the quality of evidence, and U.K. resolved the conflicts. U.K. performed the analysis. Y.K., M.M.N., F.S., and S.R. wrote the final manuscript. M.A. supervised the project. All authors have read and agreed to the final version of the manuscript.I also declare that persons who have made substantial contributions to the work reported in the manuscript, including those who provided editing and writing assistance but who are not authors, are named in the Acknowledgments section of the manuscript and have given their written permission to be named. If the manuscript does not include Acknowledgments, it is because the authors have not received substantial contributions from nonauthors.
Corresponding author
Ethics declarations
Ethics approval and consent to participate
Not applicable
Consent for publication
Not applicable
Competing interests
The authors declare no competing interests.
Additional information
Publisher’s Note
Springer Nature remains neutral with regard to jurisdictional claims in published maps and institutional affiliations.
Electronic supplementary material
Below is the link to the electronic supplementary material.
Rights and permissions
Open Access This article is licensed under a Creative Commons Attribution 4.0 International License, which permits use, sharing, adaptation, distribution and reproduction in any medium or format, as long as you give appropriate credit to the original author(s) and the source, provide a link to the Creative Commons licence, and indicate if changes were made. The images or other third party material in this article are included in the article’s Creative Commons licence, unless indicated otherwise in a credit line to the material. If material is not included in the article’s Creative Commons licence and your intended use is not permitted by statutory regulation or exceeds the permitted use, you will need to obtain permission directly from the copyright holder. To view a copy of this licence, visit http://creativecommons.org/licenses/by/4.0/. The Creative Commons Public Domain Dedication waiver (http://creativecommons.org/publicdomain/zero/1.0/) applies to the data made available in this article, unless otherwise stated in a credit line to the data.
About this article
Cite this article
Khan, U., Mubariz, M., Khlidj, Y. et al. Safety and Efficacy of Camostat Mesylate for Covid-19: a systematic review and Meta-analysis of Randomized controlled trials. BMC Infect Dis 24, 709 (2024). https://doi.org/10.1186/s12879-024-09468-w
Received:
Accepted:
Published:
DOI: https://doi.org/10.1186/s12879-024-09468-w